User talk:Hoskir: Difference between revisions
Line 5,586: | Line 5,586: | ||
* '''Seismic Details for Widening (one side):''' When widening the bridge in one direction there is not a significant benefit, and it could be detrimental, to strengthen a new wing or column while ignoring the existing structure. It may be practical to use FRP wrap to retrofit the existing columns to provide a similar level of service to a new column with seismic details, but this will likely require design computations to verify (see below). For SDC C and D, seismic details typically require a T-joint detail in the beam cap and footing, but t-joint details shall be ignored if the existing beam cap is not retrofitted. For abutments it is not practical to dig up an existing wing solely to match the new wing design so the abutment need not be designed for mass inertial forces. SPM, SLE or owner’s representative approval is required to determine the appropriate level of seismic detail implementation. | * '''Seismic Details for Widening (one side):''' When widening the bridge in one direction there is not a significant benefit, and it could be detrimental, to strengthen a new wing or column while ignoring the existing structure. It may be practical to use FRP wrap to retrofit the existing columns to provide a similar level of service to a new column with seismic details, but this will likely require design computations to verify (see below). For SDC C and D, seismic details typically require a T-joint detail in the beam cap and footing, but t-joint details shall be ignored if the existing beam cap is not retrofitted. For abutments it is not practical to dig up an existing wing solely to match the new wing design so the abutment need not be designed for mass inertial forces. SPM, SLE or owner’s representative approval is required to determine the appropriate level of seismic detail implementation. | ||
* '''Seismic Details for Widening (both sides):''' When widening in both directions the wings shall be designed to resist the mass inertial forces. Seismic details shall be added to the new columns in SDC B only if the existing columns can be retrofitted with FRP wrap to provide a similar level of service as discussed below. SDC C and D bridges may be detailed and retrofitted similar to SDC B since retrofitting the beam cap or footing is likely not practical. | * '''Seismic Details for Widening (both sides):''' When widening in both directions the wings shall be designed to resist the mass inertial forces. Seismic details shall be added to the new columns in SDC B only if the existing columns can be retrofitted with FRP wrap to provide a similar level of service as discussed below. SDC C and D bridges may be detailed and retrofitted similar to SDC B since retrofitting the beam cap or footing is likely not practical. | ||
* '''Seismic Details for Widening (FRP wrap)''': Carbon or glass fiber reinforced polymer (FRP) composite wrap should be considered to strengthen the factored axial resistance of existing columns. There are limitations to the existing and achievable column factored axial resistance with FRP wrap. The goal of the FRP wrap is to increase the factored axial resistance of the existing column to be not less than the factored axial resistance of the new column with seismic details. If an existing column cannot be retrofitted with FRP wrap to match the factored axial resistance of a new column with seismic details at the same bent then seismic details shall be ignored for all columns in the bridge substructure. See AASHTO Guide Spec for Design of Bonded FRP Systems for Repair and Strengthening of Concrete Bridge Elements, March 2023, 2nd Ed., Appendix A, Example 6 for an example for increasing column factored axial resistance with FRP wrap. Use [[751.50_Standard_Detailing_Notes# | * '''Seismic Details for Widening (FRP wrap)''': Carbon or glass fiber reinforced polymer (FRP) composite wrap should be considered to strengthen the factored axial resistance of existing columns. There are limitations to the existing and achievable column factored axial resistance with FRP wrap. The goal of the FRP wrap is to increase the factored axial resistance of the existing column to be not less than the factored axial resistance of the new column with seismic details. If an existing column cannot be retrofitted with FRP wrap to match the factored axial resistance of a new column with seismic details at the same bent then seismic details shall be ignored for all columns in the bridge substructure. See AASHTO Guide Spec for Design of Bonded FRP Systems for Repair and Strengthening of Concrete Bridge Elements, March 2023, 2nd Ed., Appendix A, Example 6 for an example for increasing column factored axial resistance with FRP wrap. Use [[751.50_Standard_Detailing_Notes#I5._Fiber_Reinforced_Polymer_(FRP)_Wrap_–_Intermediate_Bent_Column_Strengthening_for_Seismic_Details_for_Widening._Report_following_notes_on_Intermediate_bent_plan_details.|EPG 751.50 Standard Detailing Notes I5]] on plans to report factored axial resistance of existing column and new column. The flexural resistance of the column is also increased with FRP wrap, but it may not be practical to match the flexural resistance of a new column using existing longitudinal steel. For additional references, see [[751.40_LFD_Widening_and_Repair#751.40.3.2_Bent_Cap_Shear_Strengthening_using_FRP_Wrap|EPG 751.40.3.2 Bent Cap Shear Strengthening using FRP Wrap]]. | ||
Line 5,631: | Line 5,631: | ||
===I5. Fiber Reinforced Polymer (FRP) Wrap – Intermediate Bent Column Strengthening for Seismic Details for Widening. Report following notes on Intermediate bent plan details.=== | ===I5. Fiber Reinforced Polymer (FRP) Wrap – Intermediate Bent Column Strengthening for Seismic Details for Widening. Report following notes on Intermediate bent plan details.=== | ||
'''( | '''(I5.1)''' | ||
: | :Factored axial resistance of new columns = _____ kip and factored axial resistance of existing columns = _____ kip. The factored axial resistance of the existing column with FRP wrap shall not be less than the factored axial resistance of the new columns. | ||
'''( | '''(I5.2)''' | ||
:See special provisions. | :See special provisions. |
Revision as of 11:25, 31 March 2025
REVISION REQUEST 3980
620.2.16 Stop and Yield Lines (MUTCD Section 3B.16)
Guidance. Stop lines should be used to indicate the point behind which vehicles are required to stop, in compliance with a traffic control signal.
Option. Stop lines may be used to indicate the point behind which vehicles are required to stop in compliance with a STOP (R1-1) sign, a Stop Here For Pedestrians (R1-5b or R1-5c) sign, or some other traffic control device that requires vehicles to stop, except YIELD signs that are not associated with passive grade crossings.
Yield lines may be used to indicate the point behind which vehicles are required to yield in compliance with a YIELD (R1-2) sign or a Yield Here to Pedestrians (R1-5 or R1-5a) sign.
Standard. Except as provided in MUTCD Section 8B.28, stop lines shall not be used at locations where drivers are required to yield in compliance with a YIELD (R1-2) sign or a Yield Here To Pedestrians (R1-5 or R1-5a) sign or at locations on uncontrolled approaches where drivers are required by State law to yield to pedestrians.
Yield lines shall not be used at locations where drivers are required to stop in compliance with a STOP (R1-1) sign, a Stop Here For Pedestrians (R1-5b or R1-5c) sign, a traffic control signal, or some other traffic control device.
Stop lines shall consist of solid white lines extending across approach lanes to indicate the point at which the stop is intended or required.
Stop lines shall be used in advance of railroad crossings to indicate the appropriate location to stop.
When any crosswalk is installed where a permanent traffic control device is provided, such as a STOP sign or traffic signal, a stop line shall be installed in advance of the crosswalk.
Stop lines shall be 24 in. wide and shall extend across all lanes affected by the traffic control device.
Yield lines shall consist of a row of solid white isosceles triangles pointing toward approaching vehicles extending across approach lanes to indicate the point at which the yield is intended or required. The spacing of triangles in a yield line shall be consistent for that marking.
Guidance. Yield lines should be 24in. wide by 36in. long with 12 in. spacing between triangles, as shown on Standard Plan 620.00. Yield line triangles are paid for per each individual triangle. A yield line, for a lane that is 10 ft. or narrower, will consist of 4 individual triangles spaced accordingly.
Yield lines may be considered for those locations where a free right turn lane is developed but there is not an acceleration lane on the intersecting road. Yield lines may also be considered at on ramps with tapered acceleration lanes as shown in Fig. 620.2.5.3, Examples of Dotted Lined and Channelizing Line Applications for Entrance Ramp Markings.
Yield lines may also be used where engineering judgment indicates a need.
Guidance. If used, stop and yield lines should be placed a minimum of 4 ft. in advance of the nearest crosswalk line at controlled intersections, except for yield lines at roundabouts as provided for in EPG 620.3.4 Yield Lines for Roundabouts and at midblock crosswalks. In the absence of a marked crosswalk, the stop line or yield line should be placed at the desired stopping or yielding point, but should not be placed more than 30 ft. nor less than 4 ft. from the nearest edge of the intersecting traveled way. Stop lines should be placed to allow sufficient sight distance to all other approaches to an intersection.
When a stop line is used in conjunction with the STOP sign it should be placed adjacent to, or in line with, the STOP sign.
When a yield line is used in conjunction with the YIELD sign it should be placed adjacent to, or in line with, the YIELD sign.
Stop lines at midblock signalized locations should be placed at least 40 ft. in advance of the nearest signal indication.
If yield or stop lines are used at a crosswalk that crosses an uncontrolled multilane approach, the yield lines or stop lines should be placed 20 to 50 ft. in advance of the nearest crosswalk line, and parking should be prohibited in the area between the yield or stop line and the crosswalk (see Figure 620.2.17.1 Examples of Yield Lines at Unsignalized Midblock Crosswalks).
Standard. If yield (stop) lines are used at a crosswalk that crosses an uncontrolled multi-lane approach, Yield Here To (Stop Here For) Pedestrians (R1-5 series) signs (see EPG 620.2.11 Raised Pavement Markers) shall be used.
Guidance. Yield (stop) lines and Yield Here To (Stop Here For) Pedestrians signs should not be used in advance of crosswalks that cross an approach to or departure from a roundabout.
Support. Drivers yielding or stopping too close to crosswalks that cross uncontrolled multi-lane approaches place pedestrians at risk by blocking other drivers’ views of pedestrians and by blocking pedestrians’ view of vehicles approaching in the other lanes.
Option. Stop and yield lines may be staggered longitudinally on a lane-by-lane basis. Refer to "D" of Fig. 620.2.8.2.
Support. Staggered stop lines and staggered yield lines can improve the driver's view of pedestrians, provide better sight distance for turning vehicles and increase the turning radius for left-turning vehicles.
EPG 620.2.25 Stop and Yield Lines at Highway-Rail Grade Crossings contains information regarding the use of stop lines and yield lines at grade crossings.
620.2.24 Pavement Markings for Highway-Rail Grade Crossings (MUTCD Section 8B.27)
Standard. All grade crossing pavement markings shall be retroreflectorized white. All other markings shall be in accordance with EPG 620 Pavement Marking.
On paved roadways, pavement markings in advance of a grade crossing shall consist of an X, the letters RR, a no-passing zone marking (on two-lane, two-way highways with centerline markings in compliance with EPG 620.2.1), and certain transverse lines as shown in Fig. 620.2.25.1, Example of Placement of Warning Signs and Pavement Markings at Grade Crossings and Fig. 620.2.25.2, Grade Crossing Pavement Markings.
Identical markings shall be placed in each approach lane on all paved approaches to grade crossings where signals or automatic gates are located, and at all other grade crossings where the posted or statutory highway speed is 40 mph or greater.
Pavement markings shall not be required at grade crossings where the posted or statutory highway speed is less than 40 mph if an engineering study indicates that other installed devices provide suitable warning and control. Pavement markings shall not be required at grade crossings in urban areas if an engineering study indicates that other installed devices provide suitable warning and control.
Guidance. When pavement markings are used, a portion of the X symbol should be directly opposite the Grade Crossing Advance Warning sign. The X symbol and letters should be elongated to allow for the low angle at which they will be viewed.
Option. When justified by engineering judgment, supplemental pavement marking symbol(s) may be placed between the Grade Crossing Advance Warning sign and the grade crossing.
620.2.25 Stop and Yield Lines at Highway-Rail Grade Crossings (MUTCD section 8B.28)
Standard. On paved roadways at grade crossings that are equipped with active control devices such as flashing-light signals, gates, or traffic control signals, a stop line (see EPG 620.2.16) shall be installed to indicate the point behind which highway vehicles are or might be required to stop.
Guidance. On paved roadway approaches to passive grade crossings where a STOP sign is installed in conjunction with the Crossbuck sign, a stop line should be installed to indicate the point behind which highway vehicles are required to stop or as near to that point as practical.
If a stop line is used, it should be a transverse line at a right angle to the traveled way and should be placed approximately 8 ft. in advance of the gate (if present), but no closer than 15 ft. in advance of the nearest rail.
Option. On paved roadway approaches to passive grade crossings where a YIELD sign is installed in conjunction with the Crossbuck sign, a yield line (see EPG 620.2.16) or a stop line may be installed to indicate the point behind which highway vehicles are required to yield or stop or as near to that point as practical.
Guidance. If a yield line is used, it should be a transverse line at a right angle to the traveled way and should be placed no closer than 15 ft. in advance of the nearest rail (see Fig. 620.2.25.1, Example of Placement of Warning Signs and Pavement Markings at Grade Crossings).


REVISION REQUEST 3981
620.2.18 Crosswalk Markings (MUTCD Section 3B.18)
Support. Crosswalk markings provide guidance for pedestrians who are crossing roadways by defining and delineating paths on approaches to and within signalized intersections, and on approaches to other intersections where traffic stops.
In conjunction with signs and other measures, crosswalk markings help to alert road users of a designated pedestrian crossing point across roadways at locations that are not controlled by traffic control signals or STOP or YIELD signs.
At non-intersection locations, crosswalk markings legally establish the crosswalk.
Standard. When crosswalk lines are used, they shall consist of solid white lines that mark the crosswalk.
There are two styles of crosswalk markings: transverse and longitudinal (also known as continental). In most applications, the longitudinal markings are preferred and should be used to provide greater visibility, especially at midblock and uncontrolled crossings.
When used, longitudinal crosswalk markings shall be 24 inches wide and at least 6 feet in length, except that they shall be at least 8 feet in length at non-intersection crossings where the posted speed limit is 40 mph or greater.
If used, transverse crosswalk lines shall be no less than 6 inches wide and at least 6 feet apart
Guidance. Crosswalk lines, if used on both sides of the crosswalk, should extend across the full width of pavement or to the edge of the intersecting crosswalk to discourage diagonal walking between crosswalks.
At locations controlled by traffic control signals or on approaches controlled by STOP or YIELD signs, crosswalk lines should be installed where engineering judgment indicates they are needed to direct pedestrians to the proper crossing path(s).
Crosswalk lines should not be used indiscriminately. An engineering study should be performed before a marked crosswalk installed at a location away from a traffic control signal or STOP or YIELD signs. The engineering study should consider the number of lanes, the presence of a median, the distance from adjacent signalized intersections, the pedestrian volumes and delays, the average annual daily traffic (AADT), the posted or statutory speed limit or 85th-percentile speed, the geometry of the location, the possible consolidation of multiple crossing points, the availability of street lighting and other appropriate factors.
New marked crosswalks alone, without other measures designed to reduce traffic speeds, shorten crossing distances, enhance driver awareness of the crossing, and/or provide active warning of pedestrian presence, should not be installed across uncontrolled roadways where the speed limit exceeds 40 mph and either:
- A. The roadway has four or more lanes of travel without a raised median or pedestrian refuge island and an ADT of 12,000 vehicles per day or greater; or
- B. The roadway has four or more lanes of travel with a raised median or pedestrian refuge island and an ADT of 15,000 vehicles per day or greater.
Support. Chapter 4F of the MUTCD contains information on Pedestrian Hybrid Beacons. Section 4L.03 contains information regarding Warning Beacons to provide active warning of a pedestrian's presence. Section 4N.02 contains information regarding In-Roadway Warning Lights at crosswalks. Chapter 7D contains information regarding school crossing supervision.
Guidance. Because non-intersection pedestrian crossings are generally unexpected by the road user, warning signs (see Non-vehicular Sign (W11-2, W11-7)) should be installed and adequate visibility should be provided by parking prohibitions.
If used, the high-visibility longitudinal pedestrian crosswalk marking should consist of longitudinal bars 24 in. wide and spaced uniformly, centering one bar in each lane, and across each lane line, centerline, and edgeline (see Standard Plan 620.00).
When longitudinal bars are used to mark a crosswalk, the transverse crosswalk lines should be omitted. The marking design should avoid the wheel paths.
Existing 30 in. crosswalk bars should be replaced with 24 in. bars when the roadway is resurfaced.
Support. EPG 620.2.16 contains information regarding placement of stop line markings near crosswalk markings.
Option. Where permanent traffic control devices are not provided, speeds are greater than 35 mph or the crosswalk is located in rural locations where they are unexpected, the width of the crosswalk line may be increased up to 24 inches.
Crosswalks may be located mid-block if this placement offers greater safety to the pedestrian than the normal placement at an intersection. In these cases, the longitudinal bar pedestrian crosswalk marking should be used for greater emphasis and visibility. This type of marking may also be used at locations where substantial numbers of pedestrians cross without any other traffic control device, at locations where physical conditions are such that added visibility of the crosswalk is desired, or at places where a pedestrian crosswalk might not be expected.
Standard. All school crosswalks authorized by an agreement between the Commission and the school and/or city shall be marked. Crosswalks for schools shall be maintained in a manner that will provide a clearly visible marking at all times.
All school crosswalks shall be marked using both the advance school crosswalk and the school crosswalk sign, refer to EPG 903.18.8 School Sign (S1-1) and Plaques.
Option. When school crosswalks are located mid-block, the longitudinal bar pedestrian crosswalk marking should be used for greater emphasis and visibility.
Guidance. Crosswalk markings should be located so that the curb ramps are within the extension of the crosswalk markings.
Support. Detectable warning surfaces mark boundaries between pedestrian and vehicular ways where there is no raised curb. Detectable warning surfaces are required by 49 CFR, Part 37 and by the Americans with Disabilities Act (ADA) where curb ramps are constructed at the junction of sidewalks and the roadway, for marked and unmarked crosswalks. Detectable warning surfaces contrast visually with adjacent walking surfaces, either light-on-dark, or dark-on-light. The Americans with Disabilities Act Accessibility Guidelines for Buildings and Facilities (ADAAG) (see MUTCD Section 1A.11) contains specifications for design and placement of detectable warning surfaces.
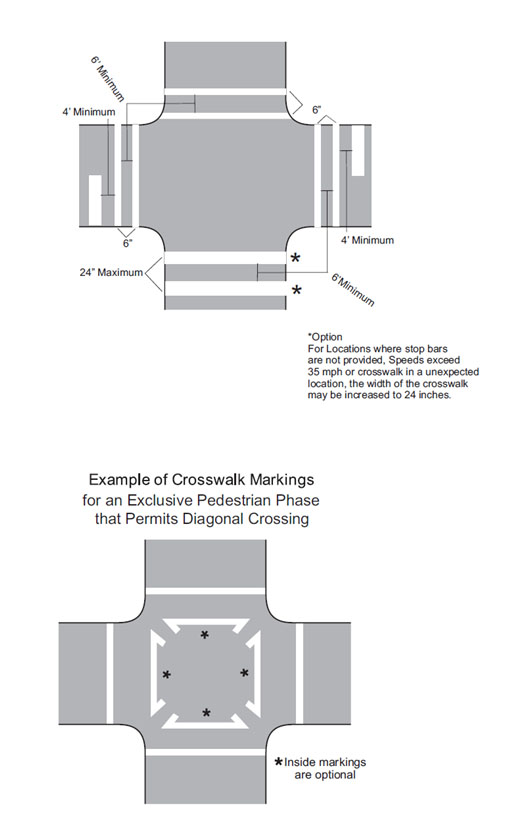
REVISION REQUEST 3997
616.6.2.2 Flags and Advance Warning Rail System on Signs
Signs may be enhanced with flags, but only during daytime hours. Flags should not be used on signs at night, except that it is allowable to leave flags on signs when the work carries over from day to night.
Standard. When standard orange flags are used in conjunction with signs, they shall not block the sign face.
616.23.1 Definitions
![]() |
Activity Area - Area of a temporary traffic control zone where work activity takes place. It is comprised of the work, traffic and buffer spaces.
Advance Warning Area - Area of a temporary traffic control zone where traffic is informed of the upcoming temporary traffic control zone.
Area Lighting - Lighting used at night to guide traffic through the temporary traffic control zone.
Annual Average Daily Traffic (AADT) - Volume of vehicular traffic using a section of highway on an average day.
Barricade - Temporary traffic control device consisting of one or three appropriately marked rails used to close, restrict or delineate all or a portion of the right of way.
Barrier-Mounted Sign - Sign mounted on a temporary or permanent traffic barrier.
Buffer Space - Area within the activity area free of equipment, material, and personnel used to provide lateral and/or longitudinal separation of traffic from the workspace or an unsafe condition.
Channelizer - Temporary traffic control device used to guide traffic or delineate an unsafe condition.
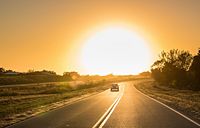
Crash Cushion - Temporary traffic control device used at fixed object and other desirable locations to reduce crash severity.
Daytime/Daylight - Period of time from one-half hour after sunrise to one-half hour before sunset.
Detour - Temporary rerouting of traffic onto an existing facility to avoid a temporary traffic control zone.
Diversion - Rerouting of traffic around an activity area using a temporary roadway or portions of an existing parallel roadway.
Divided Highway - Highway with physical separation of traffic in opposite directions.
Downstream Taper - Visual cue to traffic that access back into a closed lane is available.
Emergency Operation - Work involving the initial response to and repair/removal of safety concerns including Response Priority 1 items.
Fine Sign - Regulatory sign indicating the applicability of additional fines in a temporary traffic control zone.
Flag System – A flag bracket and two flag assemblies. Flags are used to enhance signs.
Flagger - Person who provides temporary traffic control by assigning right of way.
Flashing Arrow Panel - Temporary traffic control device with a pattern of elements capable of flashing displays (i.e. left/right arrow, double arrow, caution mode) used to provide warning or guidance to traffic.

Fleet Lighting - Rotating or flashing lights used to increase the visibility of work-related vehicles and equipment in the temporary traffic control zone.
Guide Sign - Sign showing route designations, destinations, directions, distances, services, points of interest or other geographical, recreational or cultural information.
High Speed - Posted speed of 50 mph and above.
Highway - Any facility constructed for the purposes of moving traffic.
Incident Area - Temporary traffic control zone where temporary traffic control devices are deployed in response to a traffic incident, natural disaster, special event, etc.
Intermediate-Term Stationary Operation - Daytime work occupying a location from more than one daylight period up to 3 days or nighttime work occupying a location more than 60 minutes.
Lane Taper - Temporary traffic control measure used to merge or shift traffic either left or right out of a closed lane.
Lateral Buffer Space - Obstacle-free area adjacent to the workspace or an unsafe condition that provides room for recovery of an errant vehicle.
Lighting Device - Temporary traffic control device illuminating a portion of the roadway or supplementing other traffic control devices.
Long-Term Stationary Operation - Work occupying a location longer than 3 days.
Longitudinal Buffer Space - Obstacle-free area in advance of the work space or an unsafe condition that provides room for recovery of an errant vehicle.
Low Speed - Posted speed of 45 mph and below.
Low Volume - 500 or less AADT. The rule of thumb is to count the number of vehicles passing a single reference point over a five-minute period. If not more than three vehicles pass the reference point in that period, then the road can be considered low volume for the purpose of installing work zone traffic control.
May - Indicates a permitted practice and carries no requirement or recommendation.
Mobile Operation - Work on the roadway that moves intermittently or continuously.
Motorized Traffic - Movement of vehicles and equipment on the roadway.
Multilane Highway - Highway with two or more driving lanes in the same direction of travel.
Nighttime - Period of time from one-half hour before sunset to one-half hour after sunrise.

Non-Motorized Traffic - Movement of pedestrians, bicycles, horse-drawn vehicles, etc. on roadway or within the right of way.
One-Lane, Two-Way Taper - Temporary traffic control measure used to channelize traffic through an activity area occupying one lane of an undivided, two-lane roadway.
Pavement Marking - Lines, markers, words and symbols affixed to the pavement surface to channelize and guide traffic.
Pilot Car - Vehicle used to guide a queue of vehicles through the temporary traffic control zone.
Portable Changeable Message Signs (CMS) - Temporary traffic control device capable of displaying a variety of messages to traffic.
Portable Sign - Sign mounted on temporary supports (e.g. self-driving post, easels, foldup stands, barricades, etc.).
Post-Mounted Sign - Sign mounted on a non-portable post (e.g. perforated square steel tube, u-channel, wood, etc.).
Protective Vehicle - Vehicle used to protect workers or work equipment from errant vehicles (e.g. pick up, dump truck, loader, etc.).
Regulatory Sign - Sign giving notice of traffic laws or regulations.
Roadway - Portion of highway, including shoulders, intended for use by motorized traffic.
Rural - Area generally characterized by lower volumes, higher speeds and fewer turning conflicts and conflicts with pedestrians. Includes unincorporated areas designated by community boards.
Safety Apparel - Personal protective equipment worn by a worker to improve visibility (e.g. vests, hats, etc.).
Shall - Indicates a required, mandatory, or specifically prohibitive practice. Shall statements are not to be modified or compromised based on engineering judgement or engineering study.
Short Duration Operation - Daytime or nighttime work occupying a location up to 60 minutes.
Short-Term Stationary Operation - Daytime work occupying a location more than 60 minutes, but less than 12 hours.
Should - Indicates a recommended, but not mandatory, practice in typical situations. Deviations are allowed if engineering judgement or engineering study indicates the deviation to be appropriate.
Shoulder Taper - Temporary traffic control measure used to close the shoulder.
Sign - Traffic control device conveying a static message to traffic through words or symbols.
Speed Limit - Maximum speed applicable to a section of highway as established by law.
Stop Bar - Solid white pavement marking extending across an approach lane to indicate the point where traffic is to stop.
Supplemental Warning Methods - Temporary traffic control enhancements used to increase the effectiveness of select temporary traffic control devices or the awareness of the entire temporary traffic control zone.
Taper - Series of channelizers and/or pavement markings used to move traffic into the intended path.
Temporary Traffic Barrier - Temporary traffic control device used to create a physical separation between traffic and the workspace, an unsafe condition, or non-motorized traffic.
Temporary Traffic Control Device - Item used to regulate, warn or guide traffic through a temporary traffic control zone.
Temporary Traffic Control Plan - Describes temporary traffic control measures to be used for moving traffic through a temporary traffic control zone.
Temporary Traffic Control Signal - Temporary traffic control device used to assign right of way through automatic means.
Temporary Traffic Control Zone - Section of highway where traffic conditions are changed due to a work zone or an incident area through the use of temporary traffic control devices, law enforcement or other authorized officials. It extends from the first warning sign or rotating/strobe lights on a vehicle to the last temporary traffic control device.
Termination Area - Area of a temporary traffic control zone returning traffic to the normal path.
Traffic - Highway user.
Traffic Space - Area within the activity area in which traffic is routed through the activity area.
Transition Area - Area of a temporary traffic control zone where traffic is redirected out of the normal path and into the traffic space.
Traveled Way - Portion of roadway intended for the movement of motorized traffic.
Truck-Mounted Attenuator (TMA) - Device designed to attach to the rear of protective vehicles to absorb the impact of an errant vehicle or inattentive driver.
Undivided Highway - Highway with no physical separation of traffic in opposite directions.
Urban - Area within the limits of incorporated towns and cities where the posted speed is 60 mph or less.
Vehicle-Mounted Sign - Sign mounted on a protective vehicle used in short duration and mobile operations or on a pilot car.
Warning Sign - Sign giving notice of a situation or condition that might not be readily apparent.
Work Duration - Length of time an operation occupies a location.
Work Lighting - Lighting used at night to perform activities within the workspace.
Work Location - Portion of right of way in which work is performed.
Workspace - Area within the activity area closed to traffic and set aside for workers, equipment, materials and a protective vehicle, if one is used upstream. Channelizers usually delineate workspaces.
Work Vehicle - Any vehicle by which work is performed.
Work Zone - Temporary traffic control zone where temporary traffic control devices are deployed for construction, maintenance or utility- related work activities.
Work Zone Length - Distance from last sign in the advance warning area to the last temporary traffic control device in the same direction or the last sign in the advance warning area in the opposing direction, whichever is longest.
Refer to EPG 902.18 Glossary for definitions of interchange, intersection and right of way.
616.23.2.5.1.1 Flags
Guidance is located in EPG 616.6.2.2 Flags.
616.23.2.5.1.3 Sign Design
Details, descriptions, and ordering information for signs used for temporary traffic control are specified in EPG 616.6 Temporary Traffic Control Zone Devices.
These signs may have a rigid or flexible substrate. Additional information is located in EPG 616.6.2.3 Sign Dimension and EPG 616.6.3 Sign Placement.
Flags may be used to supplement these signs provided they do not block the sign face. Additional information located in EPG 616.6.2.2 Flags.
UPLOAD NEW IMAGES
616.19.2.2.2 Sign and Flag Quality
- Acceptable Examples
-
(1)
-
(2)
-
(3)
The signs in Pictures 1, 2 and 3 are considered in good quality. Supplemental devices such as flags and/or a cone may be placed next to a sign. Picture 2 is an example of the proper placement of a FLAGGER (WO20-7) sign, with the optional flags, in advance of the hill versus after the hill. In urban areas with barrier walls and narrow shoulders, a truncated sign may be used as shown in Picture 3.
- Note: TTCDs may be highly visible during the day but may not be at night due to inadequate retroreflectivity. MoDOT and Contractor representatives should drive through the work zone at night to check nighttime visibility.
- Unacceptable Examples
-
(4)
-
(5)
-
(6)
-
(7)
-
(8)
-
(9)
Pictures 4-7 are in unacceptable condition. Dirty or damaged signs should be cleaned, repaired, or replaced before being installed. When cleaning, follow manufacturer’s recommendations, so the daytime and nighttime visibility of the sign is not adversely impacted. The MEN WORKING sign (Picture 8) should be replaced with worker symbol sign or WORKERS sign (WO-21-1 or 1a) to meet current standards. Picture 9 shows unacceptable flags, if used, deteriorated flags should be replaced.
- Unacceptable Examples
-
(10)
-
(11)
-
(12)
-
(13)
Pictures 10 - 13 are examples of unacceptable nighttime visibility. Proper storing, transporting, and covering signs is crucial to minimizing deficiencies.
REVISION REQUEST 4008
403.1.5 Mixture Production Specification Limits (Sec 403.5)
Intentional deviations from the JMF will not be permitted, except under the conditions set forth in Sec 403.11. The plant shall be operated in such a manner that the mix is produced as shown on the JMF. The specification tolerances are developed in an attempt to keep the mix as consistent as possible and to allow for some variation during production. However, these tolerances are not production limits. For example, if the target binder content is 5.0%, the binder content of the mix can range from 4.7% to 5.3% when the tolerances are applied. The contractor will not be allowed to produce the mix at 4.7% to save money.
Operating out of the specifications may reduce the contractor's pay and/or the pavement service life. When QC tests, either random or informational, are out of specification tolerances, the contractor should adjust the production to bring the mix back in. When QA tests are out of specification tolerances, the contractor should be notified immediately. The contractor is responsible for deciding when adjustments are made to control the mix. Some test properties may be allowed to deviate beyond specification limits occasionally, provided that adjustments are made and the following tests show that production is back within limits.
Production may be required to cease if the random QC or QA test results are either out of specifications far enough to indicate that the mix may be subject to failure or beyond the specification removal limits. Production should cease until verification that the problem has been corrected. An order record should be written, on the same day or the next day if paving occurs at night, describing the deficiency and the location and amount of mix affected. The contractor may elect to continue production in order to run more tests. If so, the order record should state that any mix produced after the order record was issued is at the contractor’s risk. Final disposition of the mix can then be made based on all tests and observations and may consist of acceptance at a reduced price or removal and replacement of unacceptable material.
Both QC and QA will use the following procedures to determine volumetrics of the mix and compliance with Standard Specification Sections 403.5.3 through 403.5.5. These procedures are discussed in greater detail in the Levels 1 and 2 Bituminous Training.
In situations where a retained sample must be tested, the following procedure should be used to reheat the sample. Heat the sample in an oven until the mix is workable. Take the mix out of the sample container (box, bucket, etc.) and spread it in a large pan or several smaller pans. Using this procedure, the mix will reach the molding temperature much quicker than it would if it were left in a mass in the sample container. Also, less aging of the mix occurs since the mix is in the oven for a shorter period of time. Once the mix has reached an acceptable temperature, quarter split the mix. The split portions to be used for making gyratory specimens shall then be heated to the compaction temperature. The entire suite of tests must be performed on a retained sample.
Gradation (Sec 403.5.1)
See Sieve Analysis in Plant Inspection. The gradation of the mix is not a pay factor item. However, it does have a significant influence on the volumetrics of the mix. Samples may be taken from the hot bins at a batch plant or from the combined cold feed at a drum plant. It is acceptable to determine gradation from the binder ignition sample according to AASHTO Standard Test Method T 308. Contractors should be allowed the option provided that the chosen method is spelled out in the Quality Control Plan. Gradations of extracted samples would be satisfactory as well. QC is required to sample the aggregate and perform a sieve analysis twice per lot. QA is required to independently sample the aggregate and perform a sieve analysis once per lot. These testing requirements are minimums and should be increased as necessary. Minor deviations outside the tolerances given in Standard Specification Sections 403.5.1.1 or 403.5.1.2, whichever is applicable, may be allowed if the test results indicate that the binder content, volumetrics, and density of the mix are satisfactory. If the test results are unsatisfactory, adjustments of the JMF, in accordance with Standard Specification Section 403.11, are necessary.
Stone Matrix Asphalt Tolerances (Sec 403.5.1.1)
The tolerances from the JMF for SMA mixes are given in Standard Specification Section 403.5.1.1.
Mixture Tolerance (Sec 403.5.1.2)
During production, the combined aggregate gradation must be within the following limits:
Percent Passing by Weight | |||
---|---|---|---|
Sieve Size | SP250 | SP190 | SP125 |
1 ½ in. | 100 | -- | -- |
1 in. | 90-100 | 100 | -- |
¾ in. | 92 Max. | 90-100 | 100 |
½ in. | -- | 92 Max. | 90-100 |
3/8 in. | -- | -- | 92 Max. |
#4 | -- | -- | -- |
#8 | 17-47 | 21-51 | 26-60 |
#16 | -- | -- | -- |
#30 | -- | -- | -- |
#50 | -- | -- | -- |
#100 | -- | -- | -- |
#200 | 1-7 | 2-8 | 2-10 |
Density (Sec 403.5.2)
See also Density in Plant Inspection Density Samples in Paving Operations. One sample per sublot will be taken for QC testing. QA will randomly test one of the samples from each lot to verify that a favorable comparison is obtained. These testing requirements are minimums and should be increased as necessary. SMA mixes shall have a minimum density of 94.0% with no upper limit. All other mixes shall have a density of 94.0 ±2.0%.
Shoulder Density (Sec 403.5.2.1) and Integral Shoulder (Sec 403.5.2.2)
If the shoulders and the traveled way are placed in the same pass (integrally), the cores will be taken on the traveled way. No cores will be taken on the shoulder. For example, if the paving width is 16’ with a 12’ travel lane and a 4’ shoulder, the shoulder will not be subject to density testing.
Asphalt Content (Sec 403.5.3)
QC is required to sample and test the mix for the binder content once per sublot and QA is required to independently sample and test the mix once per lot. These testing requirements are minimums and should be increased as necessary. During production, the binder content of the mix, as determined by sampling and testing, shall be within ±0.3% of the target listed on the JMF.
Voids in the Mineral Aggregate (VMA) (Sec 403.5.4)
QC is required to sample and test the mix for the VMA once per sublot and QA is required to independently sample and test the mix once per lot. These testing requirements are minimums and should be increased as necessary. The VMA of the mix shall be within –0.5% and +2.0% of the minimum required for the corresponding mix type (see Standard Specification Section 403.4.6.2).
The following table gives the ranges for each mix type:
Mix Type | VMA Limits (percent) |
---|---|
SP250 | 11.5-14.0 |
SP190 | 12.5-15.0 |
SP125 | 13.5-16.0 |
SP095 | 14.5-17.0 |
SP048 | 15.5-18.0 |
SMA | 16.5-19.0 |
Air Voids (Va) (Sec 403.5.5)
QC is required to sample and test the mix for the air voids once per sublot and QA is required to independently sample and test the mix once per lot. These testing requirements are minimums and should be increased as necessary. The Va for all mixes shall be 4.0 ±1.0%.
Tensile Strength Ratio (TSR) (Sec 403.5.6)
The TSR is used to evaluate the impact that water saturation and freeze-thaw cycles have on the strength of an asphalt mix. It can also be used to predict the susceptibility of the mix to stripping.
During production, loose mix samples will be taken and quartered as described in Mixture Production Specification Limits. TSR samples need to be taken from random locations. However, they should be taken whenever it is convenient to production, such as during a big gap between QC volumetric tests. By specification, sampling locations are from the roadway behind the paver, however, should the MoDOT inspector deem this an unsafe or impractical location, the sample may be taken from the plant. The QA sample(s) should be taken from the same point as the QC sample(s). If QC takes their sample from the plant, QA should take their sample from the plant also. This does not mean that QA should be taking their samples at the same time as QC. Two opposite quarters will be retained and the remaining two quarters will be mixed together and tested in accordance with AASHTO T283.
QC should obtain enough mix to retain a sample. QC will sample and test each mix at a minimum of once every 10,000 tons, or fraction thereof. QA will independently sample and test each mix at a minimum of once every 50,000 tons. The TSR sampling requirements are best described with an example. Suppose that 112,960 tons of SP190 are to be placed on a project. By specification, QC is required to take twelve samples and QA is required to take three samples. There are two possible scenarios for sampling this mix. QC may take eleven samples representing 10,000 tons each and a twelfth sample that represents the remaining 2,960 tons. Or QC may take ten samples that represent 10,000 tons each and two samples that represent the remaining 12,960 tons (6,480 tons each). Either scenario is acceptable. Likewise, QA may take two samples representing 50,000 tons each and a third sample that represents the remaining 12,960 tons. Or QA may take one sample that represents 50,000 tons and two samples that represent the remaining 62,960 tons (31,480 tons each). The contract quantity may be used to approximate sample 1 locations.
MoDOT should collect at least 250 pounds of asphalt mix for the QA sample, 125 pounds is retained by the RE and the other 125 pounds is sent to the Central Laboratory (typically) in 4 – 13” x 13” x 4.5” boxes for QA testing. Each box must be labeled on one side with the AASHTOWARE Project (AWP) ID, Mix Type, VMA Limits (percent) number and the mix number. An AWP record must be created for each sample, which must include all required information, the mix number, sample date, and the represented tonnage. The represented tonnage is explained in the example in the preceding paragraph. It is recommended to include the lot and sublot to the AWP record as additional information.
Additional information that may be included in the AWP record is the Gmm from the sublot that the sample was taken in (QC or QA) and the specimen weight that QC has been using. The specimen weight may be different from that shown on the JMF because of bin percent changes, etc. This information is helpful because it results in less trial-and-error for the Central Laboratory.
In the laboratory, a minimum of six specimens are compacted to a height of approximately 95 mm. The air voids of the specimens are calculated. For all mixes other than SMA, the air voids must be within 7.0 ±0.5%. For SMA mixes, the air voids must be within 6.0 ±0.5%. Half of these specimens are saturated, frozen, and thawed. These are the conditioned specimens. The degree of saturation of the conditioned specimens is also calculated. The remaining specimens are unconditioned. Then, the indirect-tensile strength of all of the specimens is determined. Therefore, the TSR is the ratio of the average tensile strength of the conditioned specimens to the average tensile strength of the unconditioned specimens.
A favorable comparison will be obtained if the QC and QA test results are within 10% of each other. The contractor’s pay will be adjusted in accordance with Standard Specification Section 403.23.5 based on the QC test results. For example, if the QC TSR is 95% and the QA TSR is 93%, a favorable comparison has been obtained and the contractor will receive a 3% bonus. However, if the difference is greater than 10%, the field office should be consulted. The field office will evaluate the air voids and saturation levels. The raw data should be collected from QC and forwarded to the field office for comparison in order to determine whether it will be necessary to proceed with 3rd party testing. QC and QA retained samples should be kept for an extended period of time so that they may be used during dispute resolution, if necessary.
The QC data should be reported in AWP (Test - SAA402AB). Contractors may report their own test results using the TSR Contractor Reporting Excel to Oracle Spreadsheet available on the MoDOT Quality Management website. Furthermore, this information is quarried regularly and, provided that a favorable comparison is reached, used to signal the appropriate time for disposal of the remaining TSR sample at the Central Lab.
Aggregate Properties (Sec 403.5.7)
The aggregate consensus tests (Fine and Coarse Aggregate Angularity, Clay Content, and Thin, Elongated Particles) are performed on the blended aggregate. The aggregate will be sampled from the combined cold feed whether dealing with a drum-mix plant or a batch plant. Aggregate samples should be taken in accordance with AASHTO R 90.
For each mix that is produced, QC shall sample the aggregate and perform the consensus tests once every 10,000 tons with a minimum of one per mix per project. QA will independently sample the aggregate and perform the consensus tests once per project. QA should also test a minimum of one QC retained sample per project. For large projects, enough QC retained samples should be tested to ensure that QC is performing the tests correctly. These testing requirements are minimums and should be increased as necessary. During production, the following tolerances are applied (see Standard Specification Sections 403.2.1 through 403.2.5 and Consensus Testing).
Property | Tolerance |
---|---|
FAA | 2% below the minimum |
CAA | 5% below the minimum |
Clay Content | 5% below the minimum |
Thin, Elongated Particles | 2% above the maximum |
Moisture Content (Sec 403.5.9)
See also Asphalt Binder Content in Plant Inspection.
Contamination (Sec 403.5.10)
See Material Acceptance in Paving Operations.
403.1.17 Quality Control (Sec 403.17)
Under QC/QA, the contractor performs quality control (QC) testing. The contractor is paid based on the results of the randomly located QC tests for Superpave mixes. Beyond random QC tests, quality control by the contractor consists of constantly monitoring materials integrity, mix production and laydown operations to ensure overall acceptability.
Asphalt Test Results (Sec 403.17.1.1)
A copy of all random QC test results shall be furnished to the QA inspector no later than the beginning of the day after testing has been performed. All raw data and printouts must be included with the testing records. Raw data consists of all weights, measurements, etc. used to arrive at the final test results. Printouts include the gyration/height data from the gyratory compactor and the asphalt content ticket from the binder ignition oven or nuclear gauge. The QC testing records must be made available to the QA inspector at all times.
It is QC’s responsibility to take appropriate action if unsatisfactory mix is being produced. This may include making adjustments to the plant to bring the mix back into specification, sampling the mix from the roadway and performing informational testing, removing mix from the roadway, etc.
Informational Tests
An informational test is a test that QC may perform between random testing to determine whether or not the mix is within specifications. Informational testing is not required and may be performed at any time and at any frequency. Generally, informational testing will be performed early in the production period. The informational test may not be completed in full. For example, QC may only compact the gyratory specimens. Doing so will yield specimen heights and the contractor may or may not make production adjustments based on these heights. Informational test samples must be clearly marked as such if they are tested and stored in the field laboratory.
QC is not required to provide the QA inspector with informational test results, since informational tests cannot be used in the QC process to determine pay factors, The timing of random number locations being given to the contractor, typically 100 to 150 tons in advance, is meant to protect the integrity of the statistical sampling process. QA always has the option of taking its own informational samples.
Informational test data may be used to determine asphalt removal limits if it is adequately documented. It should not be used for QLA under any circumstances. To be considered adequately documented the following criteria should be met:
- The gyratory pucks should be clearly identified and labeled and made available for verification.
- The gyratory printout should be available.
- The printout from the AC test should be available.
If the preceding conditions are met and the gyratory specimens are used to troubleshoot the placement, the specimens can then be weighed and bulked to determine the volumetric properties. Data from informational tests is approximate. Its only legitimate use to the QA inspector is to help determine the point on the roadway where the mixture transitioned either above or below the removal limits. We don’t want to remove acceptable mix or leave unacceptable mix in place.
Removal Limits
As an example of how informational tests may be used to designate removal limits of failing QC samples, the following situation is provided. The random QC sample shown in the diagram below fell late in sublot ‘a’ and test results indicated that voids were below the limits for removal. By specification sublot ‘a’ should be removed. By the time the test results were available and corrective action was taken, the contractor had crossed into sublot ‘b’. Assuming that mix properties were acceptable at the beginning of sublot ‘a’, the actual limits of unacceptable material are indicated by the dashed lines.
Adhering strictly to the specification, it is likely that acceptable material early in sublot ‘a’ will be removed, and it is also likely that unacceptable material early in sublot ‘b’ will be left in place. An adequately documented informational test may be used to zero in on the transitions out of, and back into, acceptable mix. It doesn’t matter that the data is approximate, only that it is above the limit for removal.
Random tests within removal limits are to be replaced by an equal number of random QC test locations, regardless of tonnage. For example, if 750 tons replace an area covered by two random tests, the new tests would be randomly chosen in each 375 ton portion of the replaced mixture.
The resident engineer has the option to determine removal limits based on puck height, provided that the informational test data is consistent with previous production.

When the random QC density core is below or above the removal limits, additional cores may be cut using the following procedure to determine the area of removal. Locations 250’ parallel to the centerline, ahead and back of the failing QC location, will be determined by the engineer. Cores will be cut in these locations and tested. If both sets of cores are not below or above the removal limits, the 500’ section will be removed and replaced with acceptable material and a new random QC core will be cut with-in the new pavement. If either set of the cores are below or above the removal limits, the whole sublot or the area in which the density core represents is subject to removal.
Any sublot of material with air voids in the compacted specimens less than 2.5 percent shall be evaluated with Hamburg testing and removed and replaced with acceptable material by the contractor if the rut depth is greater than 14.0 mm.
Inertial Profiler Test Results (Sec 610)
Surface of the pavement should be thoroughly tested with an inertial profiler or straightedge as required by Sec 610. The procedures for testing with an inertial profiler and analyzing the results with the ProVAL software program are set forth in EPG 106.3.2.59 TM-59, Determination of the International Roughness Index.
Bituminous Quality Control Plan (Sec 403.17.2)
The contractor documents the QC method with a quality control plan (QC Plan*). The QC plan for Superpave mixes shall include the contact information of the contractor’s QC representative, lot and sublot sizes and how they will be designated, the test method for determining asphalt binder content, the number of cores to be cut for density determination, and the independent third party for dispute resolution. The QC plan is approved by MoDOT Construction and Materials and used as a contract document during mix production. Contractor technicians who perform materials testing shall be certified through the MoDOT Technician Certification Program (TCP).
- Note*: A QC Plan is not required for bituminous base (BB) and pavement (BP) mixes.
Up to 3 cores are allowed at each random location, but only if spelled out in the QC plan. In the drawing below, the cylinder represents the station and offset of the random location. Best management practice is for QA to mark that location on the pavement. The first density core should have that marking on it. Any additional cores should be taken along a straight line, parallel to the centerline, within 1 foot either side of the random location.

Plant Calibration (Sec 403.17.2.2)
Retained Samples (Sec 403.17.2.3)
QC must retain the portion of each sample that is not tested after the sample has been reduced to testing size. This includes gradation, consensus, TSR, and volumetrics samples. The retained samples must be clearly identified in accordance with Standard Specification Section 403.17.2.3 and stored in the field laboratory for a minimum of 7 days. Also, all cores must be retained for a minimum of 7 days. Notwithstanding the 7 day minimum, retained samples should not be discarded until all comparison issues with the lot are resolved. If space at the field lab is an issue, the sample should be stored at the project office.
There is no legitimate reason for unidentified samples to be in the field laboratory. The QA inspector should insist that all test specimens in the field laboratory be marked as soon as they are cool enough. The identifying mark should be permanent, unique, and indicate what the sample is.
When running a QC split sample, the comparisons should be within the tolerances shown in the following table:
Loose Mix Property | Tolerance |
---|---|
Gmb | 0.010 |
Gmm | 0.010 |
AC % | 0.1% |
Gradation Sample (Sec 403.17.2.3.1)
QC will retain the portion of their gradation sample that is not tested. This includes the sample of the combined cold feed from a drum plant and all hot bin samples from a batch plant. Aggregate samples should be taken in accordance with AASHTO R 90.
Loose Mix Sample (Sec 403.17.2.3.2)
A loose mix sample consisting of roughly 100 lbs. will be taken from the roadway behind the paver, in accordance with AASHTO T168, at the required frequency. The sample will be thoroughly mixed and quartered in accordance with AASHTO R47, or with an approved splitting/quartering device. Two opposite quarters will be retained for testing during the dispute resolution process, if necessary. The remaining two quarters will be mixed together and quartered again.
The required weight of mix, as listed on the JMF, will be taken from one quarter and used to compact a specimen in accordance with AASHTO T312. The mix will be compacted to Ndes gyrations while the mix temperature is within the molding range listed on the JMF. Using the opposite quarter, follow the same procedure for the second specimen. The Gmb of each specimen will be determined and the average will be used to calculate the air voids Va and the voids in the mineral aggregate (VMA). By specification, a minimum of two compacted specimens must be used to calculate these properties.
A third quarter will be used to determine the Gmm of the mix in accordance with AASHTO T209. The minimum sample size for each type of mix can be found in the training manual. This property is used to calculate the Va and density. The volume of the sample, which is needed in the calculation, can be determined by either the weigh-in-air method or the weigh-in-water method. The weigh-in-air method consists of weighing the sample and container (with the lid) completely filled with water in air. The weigh-in-water method consists of weighing the sample and container (without the lid) completely submerged in water.
The remaining mix should be mixed together and quartered again. To determine the binder content using the nuclear gauge, enough mix should be taken from opposite quarters. The required weight of mix is listed on the JMF. A moisture content sample should be taken from the same quarters. To determine the binder content using the binder ignition oven, enough mix should be taken from one quarter. The minimum sample size for each type of mix can be found in the training manual. A moisture content sample should be taken from the same quarter. Sometimes the ignition oven may not shut itself off. The oven may be shut off manually as long as 3 consecutive readings show less than 0.01% loss. The sample should be examined to assure that a complete burn has been achieved. This will be considered a valid test.
Quality Control Laboratory (Sec 403.17.3)
The contractor is required to provide an appropriately equipped QC laboratory, however, it is not required to be at the plant. The contractor is also required to provide office space at the asphalt plant for the QA inspector to work on records and reports. Usually, these two requirements are met with one structure, but not always. The intent of the specification will be met if the QA inspector is provided with suitable facilities at the plant, but the lab is located offsite at another location, such as between the jobsite and the plant. The laboratory should have internet access in the event that cell phone service is not available.
Calibration Schedule (Sec 403.17.3.1)
Calibrations and verifications of the testing equipment are very important. If the equipment has not been calibrated or verified as required, false test results may be obtained. The maximum intervals are given in Standard Specification Section 403.17.3.1. These frequencies are taken from the AASHTO test methods and/or the manufacturer’s recommendations.
Calibration Records (Sec 403.17.3.1.2)
Periodically, the QA inspector should check the QC calibration records to ensure that the equipment has been calibrated or verified in accordance with Standard Specification Section 403.17.3.1.
REVISION REQUEST 4009
502.1.11 Contractor Quality Control (Sec 502.11)
Gradation and Deleterious Material (Sec 502.11.2.1.1)
- Aggregate Sampling Hints:
- Bin Discharge
- Ensure sampling device cuts entire stream of material
- Do not over fill the sample device
- Ensure sampling device is cleaned out
- Plant operating at usual production rates
- Obtain 3 or more equal increments
- Use AASHTO R 90
- Belt
- Sample template fits the belt
- Sweep all the fines from the belt
- Obtain 3 or more increments
- Ensure that the contractor is aware that a belt sample is being obtained
- Ensure that template is pushed all the all the way to the belt
- AASHTO R 90
- After Sampling Aggregate
- Ensure that the proper sample size was obtained
- EPG 1001.3 Sampling Procedures
- Remix material during splitting process
- MoDOT Test Method T-66
- Use AASHTO T-248 splitting procedure
- Aggregate Testing Hints
- Ensure sieves not damaged
- Ensure nesting sieve is used
- Do not over load the sieves
- Ensure sieves are cleaned
- Ensure proper test sample size used
- EPG 1001.5.1.2 Sample Preparation
- Make sure balance is calibrated and level
- Deleterious Testing Hints
- Ensure proper testing size
- For Coarse Aggregate
- EPG 1001.5.3 Percent Deleterious Substances in Coarse Aggregate
- EPG 1001.5.5 Percent Other Deleterious Substances, Clay Lumps and Shale in Fine Aggregate
- Ensure balance is calibrated and level
- Do not soak in water
- Ensure proper lighting
Moisture Content (Sec 502.11.2.1.2)
- Moisture Content Testing Hints
- Ensure balance is calibrated and level
- Use correct sample size
- Prevent loss of material when stirring
- Do not over heat sample
- Use glass plate to check for moisture
- Use air-tight container to prevent moisture loss prior to testing
Slump (Sec 502.11.2.2)
- Slump Testing Hints
- Perform test within 2 1/2 minutes
- Fill mold in 3 equal volumes
- Do not use rebar as tamper rod
- Perform on level ground
- Pre-wet equipment before testing
- Lift mold straight up
- Rod concrete properly
Entrained Air Content (Sec 502.11.2.3)
- Air Content Testing Hints
- Rod concrete properly
- Fill mold in 3 equal layers
- Perform on level ground
- Do not use rebar as tamping rod
- Use aggregate correction factor
- Tap sides of bowl after each layer
- Pre-wet equipment before testing
- Use calibrated equipment
REVISION REQUEST 4020
501.1.6 Measurement of Material (Sec 501.6)
501.1.6.1 Mass Determination (Sec 501.6.1)
The plant inspector must assure that all equipment is of an approved design and that all installations meet requirements of the specifications. There must be no attachments to scales or weighing hoppers which might hamper free movement of any part of the weighing mechanism, or cause inaccurate weighing during actual operation of the equipment.
501.1.6.2 Mixing Water (Sec 501.6.2)
Control of the amount of water added to the batch at the concrete mixer is a highly important part of the proportioning process. This is true whether water is being added through a paving mixer or is being added to central or truck mixed concrete at the plant. The inspector should be acquainted with the mechanical operation and construction of the water system. All joints should be water tight and all valves should close tightly. Leakage of water into the mixer before or after the measuring tank has been discharged should not be permitted.
501.1.6.3 Scale Calibration (Sec 501.6.3)
Scales may be calibrated in the following manner: Balance the scales accurately with no load. Use standard test weights for the test load. Test weights are suspended from the weighing hopper in such a manner that the test load is uniformly distributed. Load the aggregate scales, using combinations of weights totaling approximately 2000 pounds with test weights as required by Sec 501 of the Standard Specifications, and record scale reading. Remove weights and draw 2000 pounds of aggregate equal to the test load from the bins into the weighing hopper. Apply 2000 pounds of standard weights and record scale reading again. Repeat this procedure of drawing up aggregate, adding test weights, and recording scale weights until each scale has been calibrated to a load approximately 5% greater than the maximum working load. Cement scales should be calibrated in the same manner with approximately 500 pounds of test weights. Aggregate or cement scales may be calibrated using different weight increments, if approved by the engineer.
PCC pavement plants should be calibrated before actual proportioning starts from any new plant set up. Scale verification by the contractor or producer shall occur six months after the last plant calibration.
Calibration for other than PCC pavement plants should be at the start of the construction season. Plants located in urban areas may require more frequent calibration. Verification is required to determine if any wear and tear on the weighing equipment has occurred during the previous six months.
Check sensitivity of the scale during the calibration test by applying a small weight and observing movement of the indicator. For aggregate scales, this weight should be 5 pounds and for cement scales, 2 pounds or less. In any case, the sensitivity weight should not be greater than 0.1% of the nominal capacity of the scale. Movement on the indicator should be sufficient to indicate that the scale is out of balance.
Check the balance of each scale assembly with all weigh beams in the system free and the weight indicator counterweights moved to zero.
The inspector should check scales for balance and sensitivity of each scale assembly at random at least twice each day. These checks should be noted in the diary.
Verification of weighing equipment will consist of balancing the scales and then loading the scale to approximately 250 pounds below the scale setting, then adding approximately 500 pounds of standard test weight in not more than 150 pound increments to bring the scale to approximately 250 pounds over the scale setting.
These weight intervals for calibration, verification, balance and sensitivity are considered to be the maximum. If difficulty is encountered with the batching operation or if any of the aforementioned checks indicate excessive deviations, the plant should be recalibrated to ensure compliance.
Sec 502.4.5 of the Standard Specifications sets out certain conditions under which automatic batching equipment must be furnished. In addition to calibration procedures, automatic equipment must be checked for compliance with requirements of Sec 502.4.5 of the Standard Specifications. It is particularly important to ascertain that the discharge mechanism will not operate when ingredients have not been weighed within specified tolerances.
This check can be made by adding or removing a weight slightly greater than the permissible tolerance to see if the discharge mechanism locks and appropriate warning is given, such as a light buzzer.
In the case of a breakdown in equipment which requires a shift to manual operation, the time of breakdown should be noted in the inspector's diary. The contractor should be promptly advised of the limitation for manual batching.
Water Measuring Devices. Control of the amount of water added to the batch at the concrete mixer is a highly important part of the proportioning process. This is true whether water is being added through a paving mixer or is being added to central or truck mixed concrete at the plant. The inspector should be acquainted with the mechanical operation and construction of the water system. All joints should be water tight and all valves should close tightly. Leakage of water into the mixer before or after the measuring tank has been discharged should not be permitted.
Inspection and calibration of the water system should be performed with utmost care and thoroughness. The water measuring device must be calibrated to determine accuracy of measurements. The most common type of measuring device consists of a tank which may be emptied to various levels by adjusting the height of a movable discharge pipe inside the tank. These devices should be calibrated by weighing the amount of water discharged at various settings on the gauge dial. On some installations water may be weighed, in which case, it will be necessary to calibrate the weighing device by using standard weights. Operation of the water system during calibration should be similar to operating conditions. The full range of water measurements required during mixing operations should be covered during calibration. Several checks should be made at various settings to determine if the device will consistently measure the correct quantity within the permissible tolerances allowed by Sec 501.6 of the Standard Specifications. The water meter will be verified at the same frequency as the weighing equipment. At least one setting shall be verified within the working range.
Admixture Dispensers. All measuring devices for dispensing of admixtures should also be carefully checked. The admixture dispensers shall be calibrated by a commercial scale company, the admixture company or the concrete plant company. Admixture dispensers are usually checked by causing the dispenser to discharge into a graduate where the quantity may be accurately measured. Repeated measurements should establish that the dispenser will operate within tolerances permitted by the Standard Specifications. Results of all calibrations, verifications, and sensitivity checks should be made a part of the permanent records. Whenever the admixture dispenser is in question, the inspector has the authority to verify the dispenser.
REVISION REQUEST 4023
751.24.2.1 Design
Designs of Mechanically Stabilized Earth (MSE) walls shall be completed by consultants or contractors in accordance with Section 11.10 of LRFD specifications, FHWA-NHI-10-024 and FHWA-NHI-10-025 for LRFD. Bridge Pre-qualified Products List (BPPL) provided on MoDOT's web page and in Sharepoint contains a listing of facing unit manufacturers, soil reinforcement suppliers, and wall system suppliers which have been approved for use. See Sec 720 and Sec 1010 for additional information. The Geotechnical Section is responsible for checking global stability of permanent MSE wall systems, which should be reported in the Foundation Investigation Geotechnical Report. For MSE wall preliminary information, see EPG 751.1.4.3 MSE Walls. For design requirements of MSE wall systems and temporary shoring (including temporary MSE walls), see EPG 720 Mechanically Stabilized Earth Wall Systems. For staged bridge construction, see EPG 751.1.2.11 Staged Construction.
For seismic design requirements, see Bridge Seismic Design Flowchart. References for consultants and contractors include Section 11.10 of LRFD, FHWA-NHI-10-024 and FHWA-NHI-10-025.
Design Life
- 75 year minimum for permanent walls (if retained foundation require 100 year than consider 100 year minimum design life for wall).
Global stability:
Global stability will be performed by Geotechnical Section or their agent.
MSE wall contractor/designer responsibility:
MSE wall contractor/designer shall perform following analysis in their design for all applicable limit states.
- External Stability
- Limiting Eccentricity
- Sliding
- Factored Bearing Pressure/Stress ≤ Factored Bearing Resistance
- Internal Stability
- Tensile Resistance of Reinforcement
- Pullout Resistance of Reinforcement
- Structural Resistance of Face Elements
- Structural Resistance of Face Element Connections
- Compound Stability
- Capacity/Demand ratio (CDR) for bearing capacity shall be ≥ 1.0
- Strength Limit States:
- Factored bearing resistance = Nominal bearing resistance from Geotech report X Minimum Resistance factor (0.65, Geotech report) LRFD Table 11.5.7-1
- Extreme Event I Limit State:
- Factored bearing resistance = Nominal bearing resistance from Geotech report X Resistance factor
- Resistance factor = 0.9 LRFD 11.8.6.1
- Factored bearing stress shall be computed using a uniform base pressure distribution over an effective width of footing determined in accordance with the provisions of LRFD 10.6.3.1 and 10.6.3.2, 11.10.5.4 and Figure 11.6.3.2-1 for foundation supported on soil or rock.
- B’ = L – 2e
- Where,
- L = Soil reinforcement length (For modular block use B in lieu of L as per LRFD 11.10.2-1)
- B’ = effective width of footing
- e = eccentricity
- Note: When the value of eccentricity e is negative then B´ = L.
- Where,
- Capacity/Demand ratio (CDR) for overturning shall be ≥ 1.0
- Capacity/Demand ratio (CDR) for eccentricity shall be ≥ 1.0
- Capacity/Demand ratio (CDR) for sliding shall be ≥ 1.0 LRFD 11.10.5.3 & 10.6.3.4
- Capacity/Demand ratio (CDR) for internal stability shall be ≥ 1.0
- Eccentricity, (e) Limit for Strength Limit State: LRFD 11.6.3.3 & C11.10.5.4
- For foundations supported on soil or rock, the location of the resultant of the reaction forces shall be within the middle two-thirds of the base width, L or (e ≤ 0.33L).
- Eccentricity, (e) Limit for Strength Limit State: LRFD 11.6.3.3 & C11.10.5.4
- Eccentricity, (e) Limit for Extreme Event I (Seismic): LRFD 11.6.5.1
- For foundations supported on soil or rock, the location of the resultant of the reaction forces shall be within the middle two-thirds of the base width, L or (e ≤ 0.33L) for γEQ = 0.0 and middle eight-tenths of the base width, L or (e ≤ 0.40L) for γEQ = 1.0. For γEQ between 0.0 and 1.0, interpolate e value linearly between 0.33L and 0.40L. For γEQ refer to LRFD 3.4.
- Eccentricity, (e) Limit for Extreme Event I (Seismic): LRFD 11.6.5.1
- Note: Seismic design shall be performed for γEQ = 0.5
- Eccentricity, (e) Limit for Extreme Event II:
- For foundations supported on soil or rock, the location of the resultant of the reaction forces shall be within the middle eight-tenths of the base width, L or (e ≤ 0.40L).
- Eccentricity, (e) Limit for Extreme Event II:
General Guidelines
- Drycast modular block wall (DMBW-MSE) systems are limited to a 10 ft. height in one lift.
- Wetcast modular block wall (WMBW-MSE) systems are limited to a 15 ft. height in one lift.
- For Drycast modular block wall (DMBW-MSE) systems and Wetcast modular block wall (WMBW-MSE) systems, top cap units shall be used and shall be permanently attached by means of a resin anchor system.
- For precast modular panel wall (PMPW-MSE) systems, capstone may be substituted for coping and either shall be permanently attached to wall by panel dowels.
- For precast modular panel wall (PMPW-MSE) systems, form liners are required to produce all panels. Using form liner to produce panel facing is more cost effective than producing flat panels. Standard form liners are specified on the MSE Wall Standard Drawings. Be specific regarding names, types and colors of staining, and names and types of form liner.
- MSE walls shall not be used where exposure to acid water may occur such as in areas of coal mining.
- MSE walls shall not be used where scour is a problem.
- MSE walls with metallic soil reinforcement shall not be used where stray electrical ground currents may occur as would be present near electrical substations.
- No utilities shall be allowed in the reinforced earth if future access to the utilities would require that the reinforcement layers be cut, or if there is a potential for material, which can cause degradation of the soil reinforcement, to leak out of the utilities into the wall backfill, with the exception of storm water drainage.
- All vertical objects shall have at least 4’-6” clear space between back of the wall facing and object for select granular backfill compaction and soil reinforcement skew limit requirements. For piles, see pipe pile spacers guidance.
- The interior angle between two MSE walls should be greater than 70°. However, if unavoidable, then place EPG 751.50 J1.41 note on the design plans.
- Drycast modular block wall (DMBW-MSE) systems and Wetcast modular block wall (WMBW-MSE) systems may be battered up to 1.5 in. per foot. Modular blocks are also known as “segmental blocks”.
- The friction angle used for the computation of horizontal forces within the reinforced soil shall be greater than or equal to 34°.
- For epoxy coated reinforcement requirements, see EPG 751.5.9.2.2 Epoxy Coated Reinforcement Requirements.
- All concrete except facing panels or units shall be CLASS B or B-1.
- The friction angle of the soil to be retained by the reinforced earth shall be listed on the plans as well as the friction angle for the foundation material the wall is to rest on.
- The following requirement shall be considered (from 2009_FHWA-NHI-10-024 MSE wall 132042.pdf, page 200-201) when seismic design is required:
- For seismic design category, SDC C or D (Zones 3 or 4), facing connections in modular block faced walls (MBW) shall use shear resisting devices (shear keys, pin, etc.) between the MBW units and soil reinforcement, and shall not be fully dependent on frictional resistance between the soil reinforcement and facing blocks. For connections partially dependent on friction between the facing blocks and the soil reinforcement, the nominal long-term connection strength Tac, should be reduced to 80 percent of its static value.
- Seismic design category and acceleration coefficients shall be listed on the plans for categories B, C and D. If a seismic analysis is required that shall also be noted on the plans. See EPG 751.50 A1.1 note.
- Plans note (EPG 751.50 J1.1) is required to clearly identify the responsibilities of the wall designer.
- Do not use Drycast modular block wall (DMBW-MSE) systems in the following locations:
- Within the splash zone from snow removal operations (assumed to be 15 feet from the edge of the shoulder).
- Where the blocks will be continuously wetted, such as around sources of water.
- Where blocks will be located behind barrier or other obstacles that will trap salt-laden snow from removal operations.
- Do not use Drycast modular block wall (DMBW-MSE) systems or Wetcast modular block wall (WMBW-MSE) systems in the following locations:
- For structurally critical applications, such as containing necessary fill around structures.
- In tiered wall systems.
- For locations where Drycast modular block wall (DMBW-MSE) systems and Wetcast modular block wall (WMBW-MSE) systems are not desirable, consider coloring agents and/or architectural forms using precast modular panel wall (PMPW-MSE) systems for aesthetic installations.
- For slab drain location near MSE Wall, see EPG 751.10.3.1 Drain Type, Alignment and Spacing and EPG 751.10.3.3 General Requirements for Location of Slab Drains.
- Roadway runoff should be directed away from running along face of MSE walls used as wing walls on bridge structures.
- Drainage:
- Gutter type should be selected at the core team meeting.
- When gutter is required without fencing, use Type A or Type B gutter (for detail, see Std. Plan 609.00).
- When gutter is required with fencing, use Modified Type A or Modified Type B gutter (for detail, see Std. Plan 607.11).
- When fencing is required without gutter, place in tube and grout behind the MSE wall (for detail, see MSE Wall Standard Drawings - MSEW, Fence Post Connection Behind MSE Wall (without gutter).
- Lower backfill longitudinal drainage pipes behind all MSE walls shall be two-6” (Min.) diameter perforated PVC or PE pipe (See Sec 1013) unless larger sizes are required by design which shall be the responsibility of the District Design Division. Show drainage pipe size on plans. Outlet screens and cleanouts should be detailed for any drain pipe (shown on MoDOT MSE wall plans or roadway plans). Lateral non-perforated drain pipes (below leveling pad) are permitted by Standard Specifications and shall be sized by the District Design Division if necessary. Lateral outlet drain pipe sloped at 2% minimum.
- Identify on MSE wall plans or roadway plans drainage pipe point of entry, point of outlet (daylighting), 2% min. drainage slopes in between points to ensure positive flow and additional longitudinal drainage pipes if required to accommodate ground slope changes and lateral drainage pipes if required by design.
- Adjustment in the vertical alignment of the longitudinal drainage pipes from that depicted on the MSE wall standard drawings may be necessary to ensure positive flow out of the drainage system.
- Identify on MSE wall plans or roadway plans the outlet ends of pipes which shall be located to prevent clogging or backflow into the drainage system. Outlet screens and cleanouts should be detailed for any drain pipe.
- For more information on drainage, see Drainage at MSE Walls.
MSE Wall Construction: Pipe Pile Spacers Guidance
For bridges not longer than 200 feet, pipe pile spacers or pile jackets shall be used at pile locations behind mechanically stabilized earth walls at end bents. Corrugated pipe pile spacers are required when the wall is built prior to driving the piles to protect the wall reinforcement when driving pile for the bridge substructure at end bents(s). Pile spacers or pile jackets may be used when the piles are driven before the wall is built. Pipe pile spacers shall have an inside diameter greater than that of the pile and large enough to avoid damage to the pipe when driving the pile. Use EPG 751.50 Standard Detailing Note E1.2a on bridge plans.
For bridges longer than 200 feet, pipe pile spacers are required and the pile spacer shall be oversized to mitigate the effects of bridge thermal movements on the MSE wall. For HP12, HP14, CIP 14” and CIP 16” piles provide 24-inch inside diameter of pile spacer for bridge movement. Minimum pile spacing shall be 5 feet to allow room for compaction of the soil layers. Use EPG 751.50 Standard Detailing Note E1.2b on bridge plans.
The bottom of the pipe pile spacers shall be placed 5 ft. min. below the bottom of the MSE wall leveling pad. The pipe shall be filled with sand or other approved material after the pile is placed and before driving. Pipe pile spacers shall be accurately located and capped for future pile construction.
Alternatively, for bridges shorter than or equal to 200 feet, the contractor shall be given the option of driving the piles before construction of the mechanically stabilized earth wall and placing the soil reinforcement and backfill material around the piling. In lieu of pipe pile spacers contractor may place pile jackets on the portion of the piles that will be in the MSE soil reinforced zone prior to placing the select granular backfill material and soil reinforcement. The contractor shall adequately support the piling to ensure that proper pile alignment is maintained during the wall construction. The contractor’s plan for bracing the pile shall be submitted to the engineer for review.
Piling shall be designed for downdrag (DD) loads due to either method. Oversized pipe pile spacers with sand placed after driving or pile jacket may be considered to mitigate some of the effects of downdrag (DD) loads. Sizing of pipe pile spacers shall account for pile size, thermal movements of the bridge, pile placement plan, and vertical and horizontal placement tolerances.
When rock is anticipated within the 5 feet zone below the MSE wall leveling pad, prebore into rock and prebore holes shall be sufficiently wide to allow for a minimum 10 feet embedment of pile and pipe pile spacer. When top of rock is anticipated within the 5 to 10 feet zone below the MSE wall leveling pad, prebore into rock to achieve a minimum embedment (pile only) of 10 feet below the bottom of leveling pad. Otherwise, the pipe pile spacer requires a minimum 5 feet embedment below the levelling pad. Consideration shall also be given to oversizing the prebore holes in rock to allow for temperature movements at integral end bents.
For bridges not longer than 200 feet, the minimum clearance from the back face of MSE walls to the front face of the end bent beam, also referred to as setback, shall be 4 ft. 6 in. (typ.) unless larger than 18-inch pipe pile spacer required. The 4 ft. 6 in. dimension serves a dual purpose:
- 1) the setback ensures that soil reinforcement is not skewed more than 15° for nut and bolt reinforcement connections to clear an 18-inch inside diameter pipe pile spacers by 6 inches per FHWA-NHI-10-24, Figure 5-17C, while considering vertical and horizontal pile placement tolerances
- 2) the setback helps to reduce the forces imparted on the MSE wall from bridge movements that typically are not accounted for in the wall design and cannot be completely isolated using a pipe pile spacer. Increasing the minimum setback shall be considered when larger diameter pile spacers are required or when other types of soil reinforcement connections are anticipated
For bridges longer than 200 feet, the minimum setback shall be 5 ft. 6 in. based on the use of 24-inch inside diameter of pipe pile spacers.
If interference with soil reinforcement is not a concern and the wall is designed for forces from bridge movement, the following guidance for pipe pile spacers clearance shall be used: pipe pile spacers shall be placed 36 in. clear min. from the back face of MSE wall panels to allow for proper compaction; 12 in. minimum clearance is required between pipe pile spacers and leveling pad and 18 in. minimum clearance is required between leveling pad and pile. For isolated pile (e.g, walls skewed from the bent orientation), the pipe pile spacer may be placed 18 in. clear min. from the back face of MSE wall panels.
MSE Wall Plan and Geometrics
- A plan view shall be drawn showing a baseline or centerline, roadway stations and wall offsets. The plan shall contain enough information to properly locate the wall. The ultimate right of way shall also be shown, unless it is of a significant distance from the wall and will have no effect on the wall design or construction.
- Stations and offsets shall be established between one construction baseline or roadway centerline and a wall control line (baseline). Some wall designs may contain a slight batter, while others are vertical. A wall control line shall be set at the front face of the wall, either along the top or at the base of the wall, whichever is critical to the proposed improvements. For battered walls, in order to allow for batter adjustments of the stepped level pad or variation of the top of the wall, the wall control line (baseline) is to be shown at a fixed elevation. For battered walls, the offset location and elevation of control line shall be indicated. All horizontal breaks in the wall shall be given station-offset points, and walls with curvature shall indicate the station-offsets to the PC and PT of the wall, and the radius, on the plans.
- Any obstacles which could possibly interfere with the soil reinforcement shall be shown. Drainage structures, lighting, or truss pedestals and footings, etc. are to be shown, with station offset to centerline of the obstacle, with obstacle size. Skew angles are shown to indicate the angle between a wall and a pipe or box which runs through the wall.
- Elevations at the top and bottom of the wall shall be shown at 25 ft. intervals and at any break points in the wall.
- Curve data and/or offsets shall be shown at all changes in horizontal alignment. If battered wall systems are used on curved structures, show offsets at 10 ft. (max.) intervals from the baseline.
- Details of any architectural finishes (formliners, concrete coloring, etc.).
- Details of threaded rod connecting the top cap block.
- Estimated quantities, total sq. ft. of mechanically stabilized earth systems.
- Proposed grade and theoretical top of leveling pad elevation shall be shown in constant slope. Slope line shall be adjusted per project. Top of wall or coping elevation and stationing shall be shown in the developed elevation per project. If leveling pad is anticipated to encounter rock, then contact the Geotechnical Section for leveling pad minimum embedment requirements.
MSE Wall Cross Sections
- A typical wall section for general information is shown.
- Additional sections are drawn for any special criteria. The front face of the wall is drawn vertical, regardless of the wall type.
- Any fencing and barrier or railing are shown.
- Barrier if needed are shown on the cross section. Barriers are attached to the roadway or shoulder pavement, not to the MSE wall. Standard barriers are placed along wall faces when traffic has access to the front face of the wall over shoulders of paved areas.
Drainage at MSE Walls
- Drainage Before MSE Wall
- Drainage is not allowed to be discharged within 10 ft. from front of MSE wall in order to protect wall embedment, prevent erosion and foundation undermining, and maintain soil strength and stability.
- Drainage Behind MSE Wall
- Internal (Subsurface) Drainage
- Groundwater and infiltrating surface waters are drained from behind the MSE wall through joints between the face panels or blocks (i.e. wall joints) and two-6 in. (min.) diameter pipes located at the base of the wall and at the basal interface between the reinforced backfill and the retained backfill.
- Excessive subsurface draining can lead to increased risk of backfill erosion/washout through the wall joints and erosion at the bottom of walls and at wall terminal ends. Excessive water build-up caused by inadequate drainage at the bottom of the wall can lead to decreased soil strength and wall instability. Bridge underdrainage (vertical drains at end bents and at approach slabs) can exacerbate the problem.
- Subsurface drainage pipes should be designed and sized appropriately to carry anticipated groundwater, incidental surface run-off that is not collected otherwise including possible effects of drainage created by an unexpected rupture of any roadway drainage conveyance or storage as an example.
- External (Surface) Drainage
- External drainage considerations deal with collecting water that could flow externally over and/or around the wall surface taxing the internal drainage and/or creating external erosion issues. It can also infiltrate the reinforced and retained backfill areas behind the MSE wall.
- Diverting water flow away from the reinforced soil structure is important. Roadway drainage should be collected in accordance with roadway drainage guidelines and bridge deck drainage should be collected similarly.
- Guidance
- ALL MSE WALLS
- 1. Appropriate measures to prevent surface water infiltration into MSE wall backfill should be included in the design and detail layout for all MSE walls and shown on the roadway plans.
- 2. Gutters behind MSE walls are required for flat or positive sloping backfills to prevent concentrated infiltration behind the wall facing regardless of when top of backfill is paved or unpaved. This avoids pocket erosion behind facing and protection of nearest-surface wall connections which are vulnerable to corrosion and deterioration. Drainage swales lined with concrete, paved or precast gutter can be used to collect and discharge surface water to an eventual point away from the wall. If rock is used, use impermeable geotextile under rock and align top of gutter to bottom of rock to drain. (For negative sloping backfills away from top of wall, use of gutters is not required.)
- District Design Division shall verify the size of the two-6 in. (min.) diameter lower perforated MSE wall drain pipes and where piping will daylight at ends of MSE wall or increase the diameters accordingly. This should be part of the preliminary design of the MSE wall. (This shall include when lateral pipes are required and where lateral drain pipes will daylight/discharge).
- BRIDGE ABUTMENTS WITH MSE WALLS
- Areas of concern: bridge deck drainage, approach slab drainage, approach roadway drainage, bridge underdrainage: vertical drains at end bents and approach slab underdrainage, showing drainage details on the roadway and MSE wall plans
- 3. Bridge slab drain design shall be in accordance with EPG 751.10.3 Bridge Deck Drainage – Slab Drains unless as modified below.
- 4. Coordination is required between the Bridge Division and District Design Division on drainage design and details to be shown on the MSE wall and roadway plans.
- 5. Bridge deck, approach slab and roadway drainage shall not be allowed to be discharged to MSE wall backfill area or within 10 feet from front of MSE wall.
- (Recommended) Use of a major bridge approach slab and approach pavement is ideal because bridge deck, approach slab and roadway drainage are directed using curbs and collected in drain basins for discharge that protect MSE wall backfill. For bridges not on a major roadway, consideration should be given to requiring a concrete bridge approach slab and pavement incorporating these same design elements (asphalt is permeable).
- (Less Recommended) Use of conduit and gutters:
- Conduit: Drain away from bridge and bury conduit daylighting to natural ground or roadway drainage ditch at an eventual point beyond the limits of the wall. Use expansion fittings to allow for bridge movement and consider placing conduit to front of MSE wall and discharging more than 10 feet from front of wall or using lower drain pipes to intercept slab drainage conduit running through backfill.
- Conduit and Gutters: Drain away from bridge using conduit and 90° elbow (or 45° bend) for smoothly directing drainage flow into gutters and that may be attached to inside of gutters to continue along downward sloping gutters along back of MSE wall to discharge to sewer or to natural drainage system, or to eventual point beyond the limits of the wall. Allow for independent bridge and wall movements by using expansion fittings where needed. See EPG 751.10.3.1 Type, Alignment and Spacing and EPG 751.10.3.3 General Requirements for Location of Slab Drains.
- 6. Vertical drains at end bents and approach slab underdrainage should be intercepted to drain away from bridge end and MSE wall.
- 7. Discharging deck drainage using many slab drains would seem to reduce the volume of bridge end drainage over MSE walls.
- 8. Drain flumes at bridge abutments with MSE walls do not reduce infiltration at MSE wall backfill areas and are not recommended.
- DISTRICT DESIGN DIVISION MSE WALLS
- Areas of concern: roadway or pavement drainage, MSE wall drainage, showing drainage details on the roadway and MSE wall plans.
- 9. For long MSE walls, where lower perforated drain pipe slope become excessive, non-perforated lateral drain pipes, permitted by Standard Specifications, shall be designed to intercept them and go underneath the concrete leveling pad with a 2% minimum slope. Lateral drain pipes shall daylight/discharge at least 10 ft. from front of MSE wall. Screens should be installed and maintained on drain pipe outlets.
- 10. Roadway and pavement drainage shall not be allowed to be discharged to MSE wall backfill area or within 10 feet from front of MSE wall.
- 11. For district design MSE walls, use roadway or pavement drainage collection pipes to transport and discharge to an eventual point outside the limits of the wall.
- Example: Showing drain pipe details on the MSE wall plans.
-
ELEVATION SHOWING DRAIN PIPE
-
Alternate option
-
Section A-A
Notes: |
E1. Excavation and Fill
(E1.1) Use when specified on the Design Layout.
- Existing roadway fill under the ends of the bridge shall be removed as shown. Removal of existing roadway fill will be considered completely covered by the contract unit price for roadway excavation.
Use one of the following two notes where MSE walls support abutment fill.
(E1.2a) [MS Cell] Use when pipe pile spacers are shown on plan details and bridge is 200 feet long or shorter. Add “See special provisions” to the pipe pile spacer callout and add table near the callout.
See special provisions.
Pile Encasement | Option Used (√) |
---|---|
Pipe Pile Spacer | |
Pile Jacket |
MoDOT Construction personnel will indicate the pile encasement used.
(E1.2b) Use note when pipe pile spacers are shown on plan details for HP12, HP14, CIP 14” and CIP 16” piles and bridge is longer than 200 feet. For larger CIP pile size modify following note and use minimum 6” larger pipe pile spacer diameter than CIP pile.
The pipe pile spacers shall have an inside diameter equal to 24 inches.
(E1.4) Use for fill at pile cap end bents. Use the first underlined portion when MSE walls are present. Use approach for semi-deep abutments.
- Roadway fill, exclusive of Select Granular Backfill for Structural Systems, shall be completed to the final roadway section and up to the elevation of the bottom of the concrete approach beam within the limits of the structure and for not less than 25 feet in back of the fill face of the end bents before any piles are driven for any bents falling within the embankment section.
REVISION REQUEST 4028
751.5.9.2.8 Development and Lap Splices
Development and Lap Splice Table of Contents |
---|
1. General |
2. Development and Lap Splices of Straight Deformed Bars in Tension |
3. Development and Lap Splices of Deformed Bars in Compression |
4. Development and Lap Splices of Standard Hooked Deformed Bars in Tension |
751.5.9.2.8.1 Development and Lap Splice General
Development of Straight Tension Reinforcement
Development lengths for tension reinforcement shall be calculated in accordance with LRFD 5.10.8.2.1.
Excess reinforcement modification factor (λer) and beneficial clamping stresses (βt component of λrc) of LRFD 5.10.8.2.1c may be used in situations where development length is difficult to attain. All other modification factors shall be used.
Temperature and shrinkage reinforcement are assumed to fully develop the specified yield stresses. Therefore the development length shall not be reduced by λer .
Development lengths for tension reinforcement have been tabulated on the following pages and include the modification factors except as described above.
Lap Splices of Tension Reinforcement (Straight and Hooked)
Lap splice lengths for tension reinforcement shall be calculated in accordance with LRFD 5.10.8.4.2a and 5.10.8.4.3a. Class B splices are preferred when possible, however it is permissible to use Class A when physical space is limited and Class A requirements are met. It should be noted that "required by analysis" of the Class A requirements is based on the stress encountered at the splice location, which is not necessarily the maximum stress used to design the reinforcement. Lap splice lengths for tension reinforcement have been tabulated on the following pages and include the development length modification factors as described above.
Development of Hooked Tension Reinforcement
Development lengths of hooked tension reinforcement shall be calculated in accordance with LRFD 5.10.8.2.4.
Excess reinforcement modification (λer) and beneficial clamping stresses (βt component of λrc) of LRFD 5.10.8.2.1c may be used in situations where development length is difficult to attain. The permissible 20 percent reduction of LRFD 5.10.8.2.4c may be used in situations where development length is difficult to attain and where required conditions are met. All other modification factors shall be used.
Development lengths of hooked tension reinforcement have been tabulated on the following pages and include the modification factors except as described above.
Development of Compression Reinforcement
Development lengths for compression reinforcement shall be calculated in accordance with LRFD 5.10.8.2.2.
Excess reinforcement modification factor (λer) of LRFD 5.10.8.2.2b may be used in situations where development length is difficult to attain. All other modification factors shall be used.
Development lengths for compression reinforcement have been tabulated on the following pages and include the modification factors except as described above.
Lap Splices of Compression Reinforcement
Lap splices lengths for compression reinforcement shall be calculated in accordance with LRFD 5.10.8.4.2a and 5.10.8.4.5a.
Splice lengths for compression reinforcement have been tabulated on the following pages.
751.5.9.2.8.2 Development and Lap Splices of Straight Deformed Bars in Tension
The values in the following table are based on Grade 60 bars (ƒy = 60 ksi) and may be adjusted for yield strengths up to 100 ksi. The final step in the table adjusts values for other material strengths. The values for Grade 40 bars are 45% (402/602) of the values in the table (not less than 12 inches), and values for 100 ksi bars are 280% (1002/602) of the values in the table.
751.5.9.2.8.3 Development and Lap Splices of Deformed Bars in Compression
The values in the following table are based on Grade 60 bars. Development lengths may be adjusted for yield strengths up to 100 ksi. Lap splice lengths for yield strengths greater than 60 ksi up to 100 ksi shall be calculated in accordance LRFD 5.10.8.4.5a. The final step in the table adjusts values for other material strengths. The values for Grade 40 bars are 40/60 of the values in the table (not less than 8 in. for development length and 12 in. for lap splice length).
751.5.9.2.8.4 Development and Lap Splices of Standard Hooked Deformed Bars in Tension
The hooked bar development length (ldh) is measured from the critical section to the outside edge of the hook.
The values in the following table are based on Grade 60 bars. Due to the complexity of the ldh formula, hooked bar development lengths will need to be calculated manually for ƒc other than 3 and 4 ksi and for ƒy other than 60 ksi. Transverse reinforcement requirements for other material strengths are specified at the bottom of the table.
751.8.3.2 Steel Reinforcement
Barrel Section
Standard boxes shall have main reinforcement placed perpendicular to the centerline of culvert. In any case, main reinforcement should not be skewed more than 25° from a line normal to the centerline of the culvert. (See LRFD 9.7.1.3.) The bar sizes, spacings and lengths given in the Standard Plans 703.17, 703.47 and 703.87 are applicable for uncoated steel reinforcement. Figure 751.8.3.2.1 shows a typical cross-section of standard box culvert and bar marks of steel reinforcement which are described below:
A1 bar - Steel reinforcement shall be designed for maximum positive moment in the top slab. This bar is placed transversely perpendicular to the centerline of culvert at the bottom of top slab. Place A1 bars into headwall or edge beam as close as practical.
A2 bar - Steel reinforcement shall be designed for maximum positive moment in the bottom slab. This bar is placed transversely perpendicular to the centerline of culvert at the top of bottom slab.
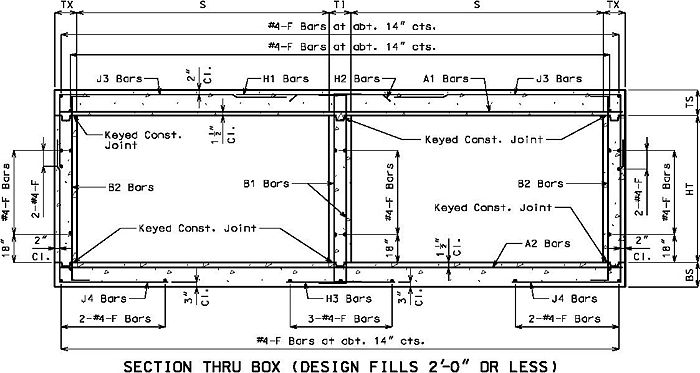
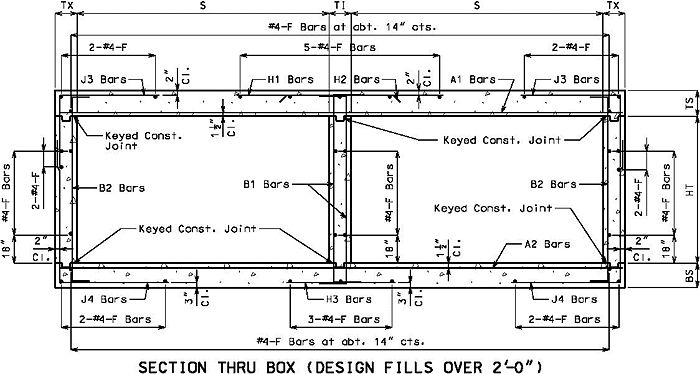
B1 bar - Steel reinforcement shall be designed for maximum combined axial load and moment at interior walls. This bar is placed vertical near stream faces of the wall. Minimum steel reinforcement of #5 bars spaced at 12” centers shall be provided. This bar should be extended into the top and bottom slabs. A hook bar may be required if the embedment length is insufficient due to slab thickness limitations. [[751.5 Standard Details#751.5.9.2.8.1 Development and Lap Splice General|EPG 751.5.9.2.8.1 Development and Lap Splice General] has information pertaining to development of tension reinforcement and hooks.
B2 bar – For culverts with bottom slabs, steel reinforcement shall be designed for the maximum positive moment in the exterior wall. For culverts on rock, steel reinforcement shall be designed for the maximum combined positive moment and axial load. This bar is placed vertical near the stream face of the wall. Minimum steel reinforcement of #5 bar spacing at 12” centers shall be provided. This bar should be extended into the top and bottom slabs. A hook bar may be required if the embedment length is insufficient due to slab thickness limitations. EPG 751.5.9.2.8.1 Development and Lap Splice General has information pertaining to development of tension reinforcement and hooks.
J3 bar - Steel reinforcement shall be designed for the maximum negative moment in the top corner of the culvert. This bar is placed vertical along the wall and transversely perpendicular to the centerline of culvert.
J4 bar - Steel reinforcement shall be designed for maximum negative moment in the bottom corner of the culvert. The J4 bar should also be designed for the maximum negative moment near the mid-height of the exterior wall. This bar is placed vertical along the wall and transversely perpendicular to the centerline of culvert.
H1 bar - Steel reinforcement shall be designed for the maximum negative moment in the top slab over the interior walls. This bar is placed transversely perpendicular to the centerline of culvert at the top of top slab. Its spacing is alternated with spacing of H2 bar. The length of H1 bar is longer than the length of H2 bar.
H2 bar - Steel reinforcement shall be designed for the maximum negative moment in the top slab over the interior walls. This bar is placed transversely perpendicular to the centerline of culvert at the top of top slab. Its spacing is alternated with spacing of H1 bar.
F bar - Longitudinal steel reinforcement provides for temperature and shrinkage control. Use #4 bars at about 14” centers for all interior faces. A minimal number of longitudinal bars in exterior faces are also provided primarily to aid in construction. This bar is placed parallel to the centerline of culvert. Additional longitudinal reinforcement may be required to provide for lateral distribution of concentrated live loads. For distribution of reinforcement, see EPG 751.8.2.6.
Headwalls and Edge Beams
Figure 751.8.3.2.2 shows a typical cross-section through headwalls and edge beams, and bar marks of steel reinforcement which are described below. The reinforcement values given below shall be considered standard for headwalls and minimum recommended values for edge beams.
If at least the minimum headwall dimensions are provided (see Fig. 751.8.3.2.2) the steel reinforcement in the top slab need not be increased over that required for barrel design. Otherwise, the width of the edge beam shall be taken as 3 feet and additional reinforcement in the top and bottom of slab is required.
D1 bar – Place 2- #8 bars at the top of headwalls or edge beams. These bars shall be placed along the headwall or edge beam.
D2 bar – Place these bars between D1 bars at the top of headwalls or edge beam and centered over interior walls. The total length of the bar is equal to two times larger value of 48 bar diameters or ¼ clear span length of headwall or edge beam. Neglect this bar for single span and if clear span length along headwall is less than or equal to 10’ for multiple spans. Otherwise, use a number of bars and sizes as indicated below:
- 2- #8 bars when 10’ 13’
- * 2- #9 bars when 13’
* The required area of steel reinforcement should be checked if clear span length along edge beam exceeds 20’.
H bar - Provide 4- #8 bars at bottom of headwalls or edge beam when edge beam is skewed. These bars shall be placed along the headwall or edge beam.
R1 bar – Provide minimum #5 bar spacing at 12” centers. This bar is placed perpendicular to longitudinal axis of upstream headwall or edge beam.
R2 bar - Provide minimum #5 bar spacing at 12” centers. This bar is placed perpendicular to longitudinal axis of upstream headwall or edge beam.
R3 bar - Provide minimum #5 bar spacing at 12” centers. This bar is placed perpendicular to longitudinal axis of downstream headwall or edge beam.

Wings
F bar - Longitudinal steel reinforcement provides for temperature and shrinkage control. Use #4 bars at about 14” centers for all interior faces. A minimal number of longitudinal bars in exterior faces are also provided primarily to aid in construction. This bar should be placed longitudinal along wing walls as shown in Figure 751.8.3.2.3. For wings on rock, longitudinal F bars should be designed using maximum moment and shear as specified in EPG 751.8.2.5.
G bar – Provide the same bar size and spacing as B1 or B2 bar for interior (Figure 751.8.3.2.3(b)) or exterior wall (Figure 751.8.3.2.3(a)), respectively.
J1 or J6 bar – Provide 2- #7 bars at each face of wing walls. These bars are provided for edge beam action and for support in extreme event scenarios, such as washout. The J6 callout is used for flared wings.
J5 bar – Steel reinforcement shall be designed for moment and shear based on Coulomb or Rankine active earth pressure. In any case, the provided steel area of J5 bar shall not be less than that provided by the adjoined wall.
Toe Walls
E1 bar – Provide 4- #5 bars and they should extend into wing walls as far as practical as shown in Figure 751.8.3.2.3. For wing walls on rock, these bars shall be extended 12” into the rock and grouted.
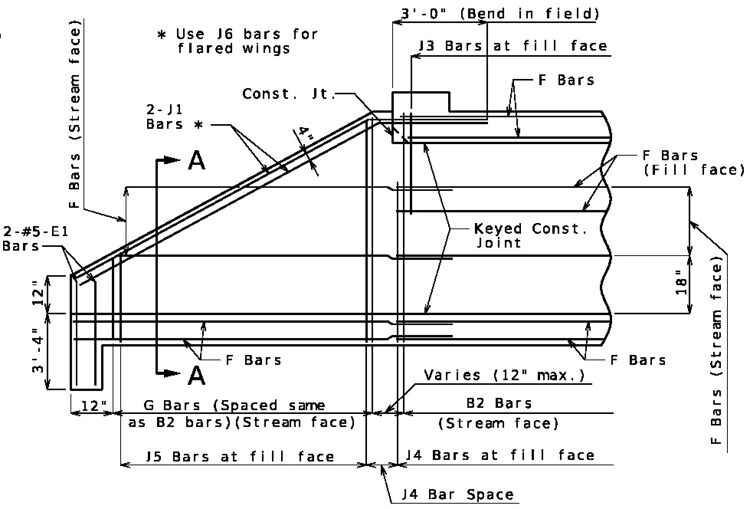


Collar Beams
Figure 751.8.3.2.4 shows steel reinforcement details of collar beams. The figure also shows that two layers of roofing felt shall be provided between culvert and collar beams. This will allow free lateral movement of adjoined sections.

-
(b)

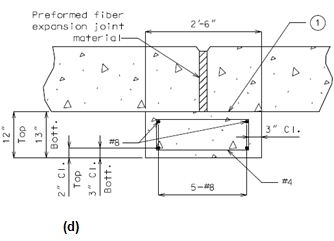
For box culverts where collars are required and the precast option is used, precast concrete box culvert ties in accordance with Sec 733 and Std. Plan 733.00 shall be provided between all precast sections. |
Reinforcement Concrete Cover
The minimum concrete cover shall be 1-1/2” (clear) except the following:
- Top Slab
- The minimum concrete cover shall be 2” (clear) at top and 1-1/2” (clear) at bottom of the slab.
- Bottom Slab
- The minimum concrete cover shall be 1-1/2” (clear) at top and 3” (clear) at bottom of the slab.
- Walls and Wing Walls
- The minimum concrete cover shall be 2” (clear) at fill face and 1-1/2” (clear) at stream face.
- Wearing Surface
- A 1” monolithic protective surface shall be used on the bottom of bottom slab to compensate for pouring concrete on uneven earth surfaces. In special cases, where abrasion on the stream faces is a concern, a 1/2" monolithic wearing surface may be used on stream faces of walls and bottom slab. In the analysis, the protective surface and wearing surfaces (when considered) are included as part of the member thickness, but shall be excluded in the calculation of effective depth of the member for design.
751.10.1.14 Girder and Beam Haunch Reinforcement
General
- Steel Beams and Girders
- Haunch reinforcement consisting of #4 hairpin bars shall be provided where the embedment of existing studs into a new slab is less than 2 inches or for an excessive haunch where at centerline of beam or girder exceeds 3 inches.
- Prestressed Beams or Girders with Full Depth CIP Decks (Conventional or SIP forms)
- Haunch reinforcement consisting of #4 hairpin bars shall be provided when haunch at centerline of beam or girder exceeds:
- 3 inches for Type 2, 3, 4 girders
- 4 inches for Type 6, 7 and 8 girders (bulb-tee), NU girders and spread beams
- Prestressed Beams or Girders and Partial Depth CIP Decks (Prestressed Panels)
- Haunch reinforcement should not be required with precast prestressed panel decks due to joint filler limits.
Details
When possible, hairpin bars and tie bars shall be clearly shown on the section thru slab; otherwise, a part section showing hairpins shall be provided. Include these bars in the slab reinforcing steel quantities.

- (1) Top of slab to bottom of longitudinal bars.
- (2) Haunch limit specified above.
- (3) Use tie bars at the discretion of the Structural Project Manager or the Structural Liaison Engineer.
- (4) The bottom longitudinal bars should be shown to be used as tie bars or add a note allowing adjustment.
- (5) Add asterisked note when there is insufficient clearance or hairpins with varying vertical heights may be used.
Hairpin bars and tie bars shall be shown on the plan of slab. Splice lengths of the tie bars shall also be specified if required (19” for #4 bars). For deck replacements without a plan of slab the hairpin bars and tie bars shall be shown either on a part plan detail or in a table. Include these bars in the slab reinforcing steel quantities.

Hairpin bars and tie bars shall be included in the bill of reinforcing. Include these bars in the slab reinforcing steel quantities.
![]() |
“C” is based on the top horizontal legs located above the longitudinal bars of the bottom mat at the location of the maximum haunch. |
751.12.1.2.7 Details of Mounting Light Poles on Safety Barrier Curbs
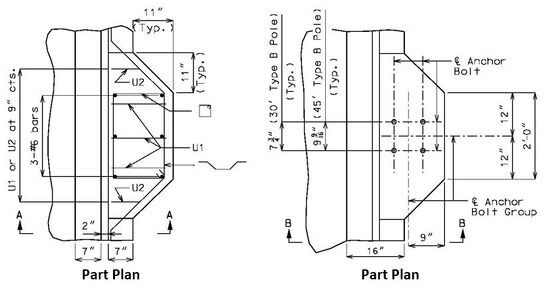


Anchor bolts and nuts shall be in accordance with ASTM F1554 Grade 55. Anchor bolts, nuts and washers shall be fully galvanized, See 751.50.H4.2.2 for additional information.
Note to Detailer: Extend slab transverse steel to edge of slab in blister region often shown with an additional detail with the slab details.
Note: Conduit not shown for clarity.
751.12.1.3.2 Typical Section Reinforcement
The single R bar adds to the rigidity of the reinforcement during construction and it is believed to help prevent cracking. The single bar also appears to assist maintaining uniform reinforcement cover.
Splice length for epoxy coated horizontal #5 bars in barrier shall be 30 inches (25” for galvanized bars).
All bent bars are formed using stirrup bends except for the Type D #5-R1 bars.
All values may be used with both 2.0% and 3/16 inch-per-foot cross slopes.

751.12.1.3.3.1 Type D Ending on Integral End Bents
Use when distance between upper and lower construction joint in wings is at least 25½ inches.
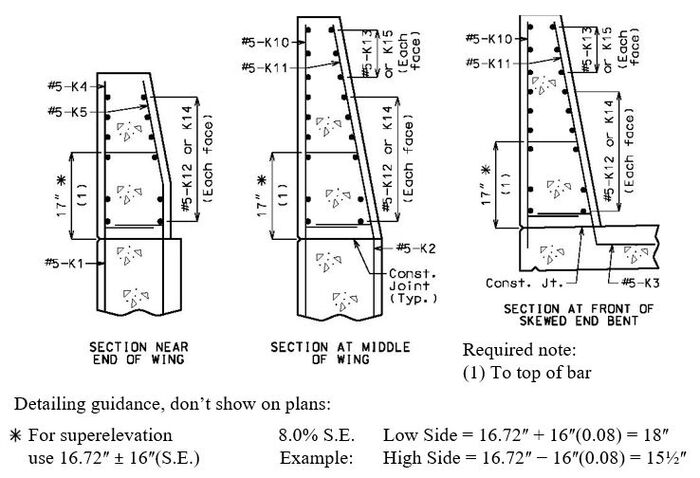


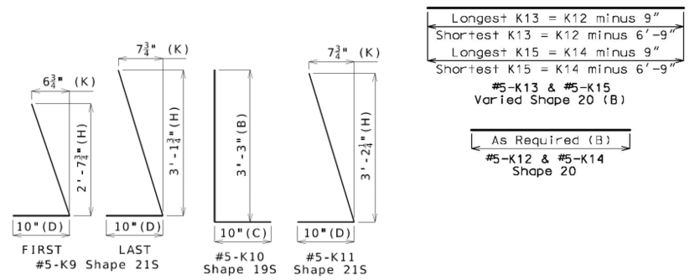
751.12.1.3.3.2 Type H Ending on Integral End Bents
Use when distance between upper and lower construction joint in wings is at least 25½ inches.
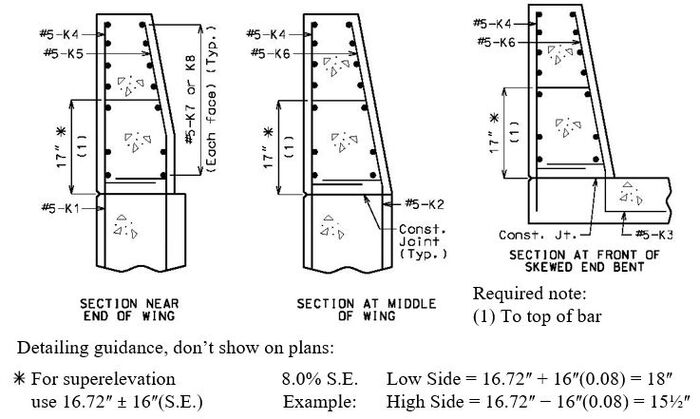

751.12.1.3.3.3 Type D Ending on Shallow Integral End Bents
Use when distance between upper and lower construction joint in wings is less than 25½ inches.
Formulas extend bars to within 1½ʺ of lower construction joint.
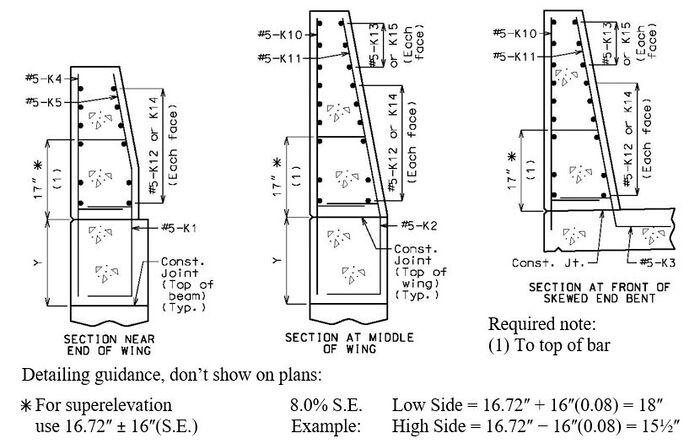


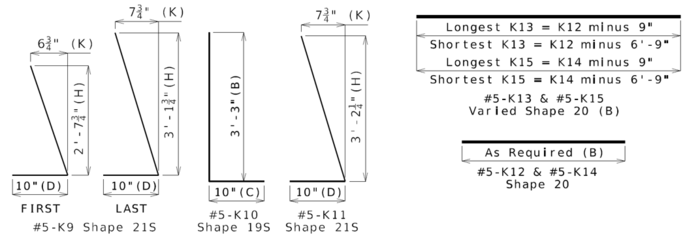
751.12.1.3.3.4 Type H Ending on Shallow Integral End Bents
Use when distance between upper and lower construction joint in wings is less than 25½ inches.
Formulas extend bars to within 1½ʺ of lower construction joint.
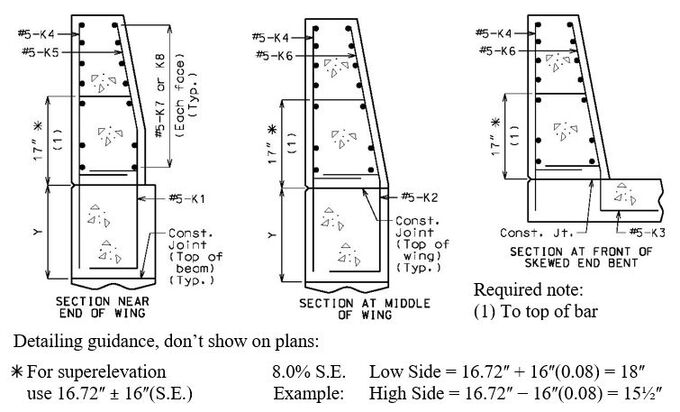

751.12.1.3.3.5 Type D Ending on Non-Integral End Bents
Use when distance between upper and lower construction joint in wings is at least 25½ inches.
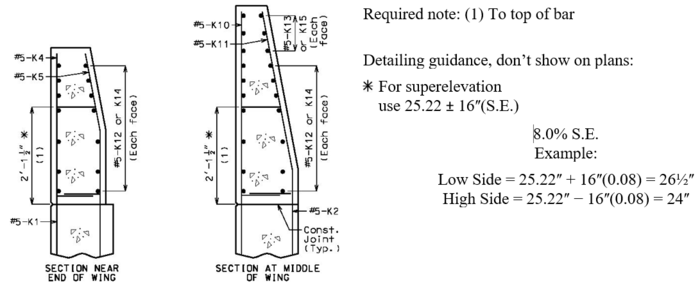


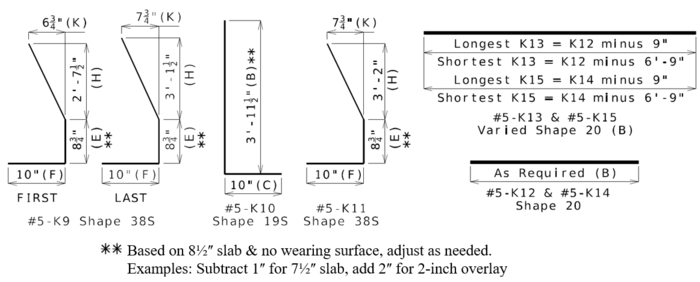
751.12.1.3.3.6 Type H Ending on Non-Integral End Bents
Use when distance between upper and lower construction joint in wings is at least 25½ inches.

751.12.1.3.3.7 Type D Ending at End of Slab (Redecks)
Splice length of epoxy coated #5 K12 and #5 K13 bars with #5 R-bars shall be 30 inches (25 inches for galvanized bars).

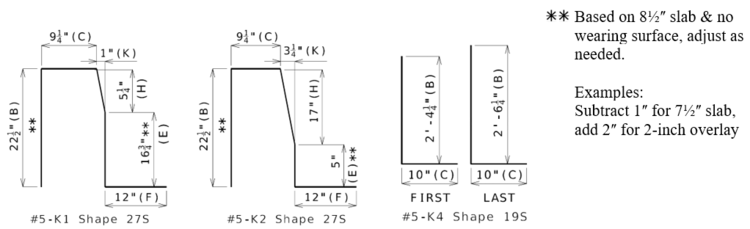


751.12.1.3.3.8 Type H Ending at End of Slab (Redecks)
Splice length of epoxy coated #5 K7 bars with #5 R-bars shall be 30 inches (25 inches for galvanized bars).
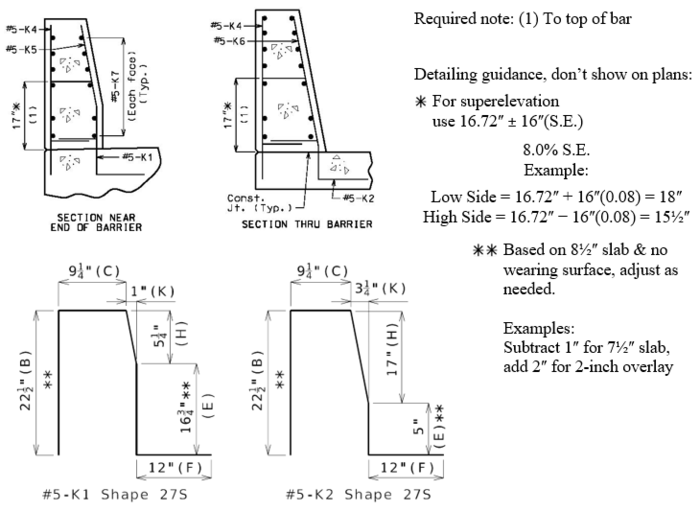

751.12.1.4.2 Typical Section Reinforcement
The single R bar adds to the rigidity of the reinforcement during construction and it is believed to help prevent cracking. The single bar also appears to assist maintaining uniform reinforcement cover.
Splice length for horizontal epoxy coated #5 bars in barrier curb shall be 30 inches (25 inches for galvanized bars).
All bent bars are formed using stirrup bends except for the R1 bars.
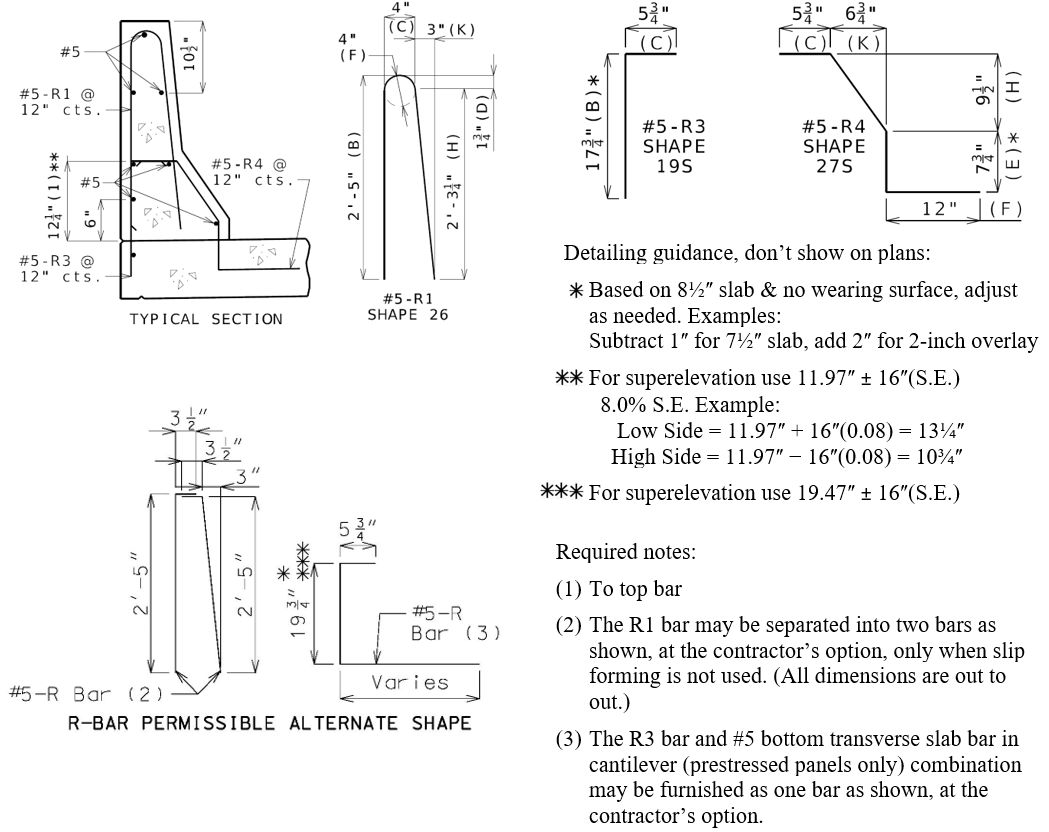
751.12.1.4.3 End of Barrier Reinforcement
See barrier ending on end bents sheets of the barrier standard drawings for the required details. The bars shown below are for barrier ending on wing walls; see barrier ending at end of slab sheet of the barrier standard drawings for reinforcement details for barrier ending on slabs.
Splice length of #5-K9 bars with #4 K-bars above wing walls shall be 31 inches (embedment of #5 bars controls over splice length of #4 bars).
All bent bars are formed using stirrup bends except for the K4 and K11 bars.
Ending on Integral End Bents and Semi-Deep Abutments
Use when distance between upper and lower construction joint in wings is at least 25½ inches.



- * On skewed integral end bents, if the end K3 bars do not meet the minimum 1 1/2" clearance from the front face of the diaphragm, a K12 bar shall be substituted.
- * Based on no wearing surface, adjust as needed. Example: Add 2ʺ for 2ʺ W.S.
- * Also based on 8½ʺ slab, adjust as needed. Example: Subtract 1ʺ for 7½ʺ slab
Ending on Shallow Integral End Bents
Use when distance between upper and lower construction joint in wings is less than 25½ inches.
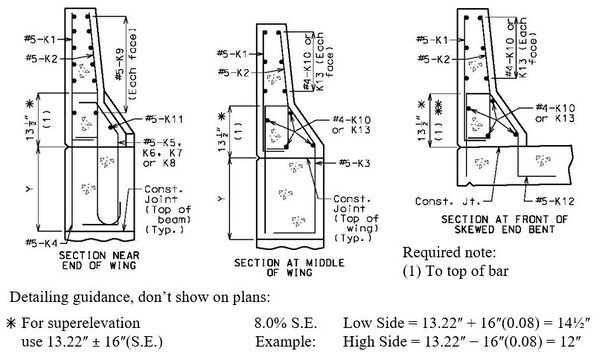
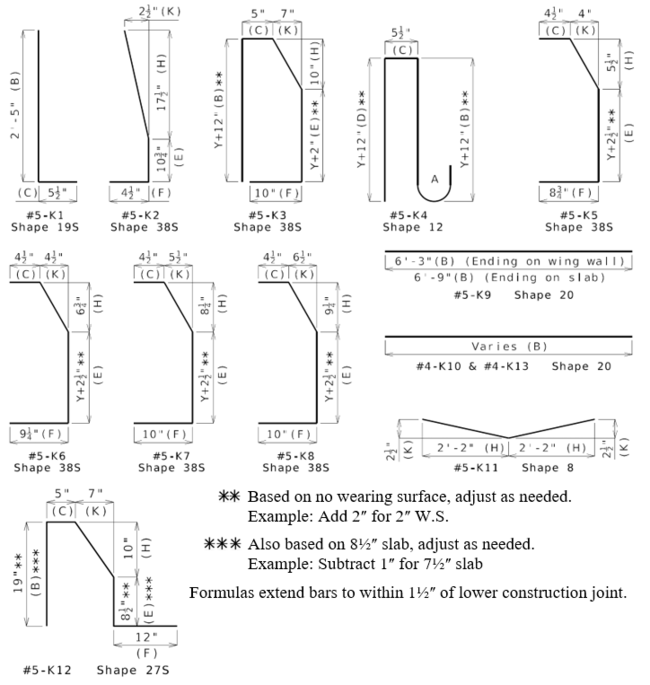
Ending on Non-Integral End Bents
Use when distance between upper and lower construction joint in wings is at least 25½ inches.



751.12.1.6 Type A (32ʺ New Jersey Shaped Median)
Note: Use same grade reinforcing steel in barrier as in slab.
- Splice length for epoxy coated #5-R bars in barrier shall be 30 inches (25 inches for galvanized bars).
- Do not use this barrier over precast prestressed panels.

Twin Bridge Median Barrier Details
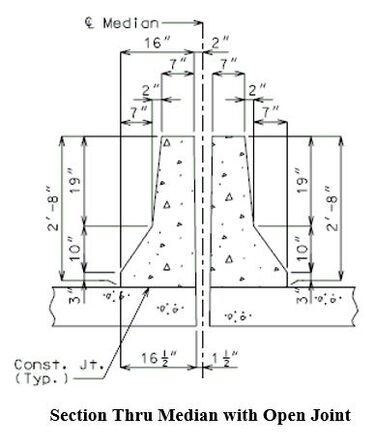
751.21.3.3.1 Spread Box Beams

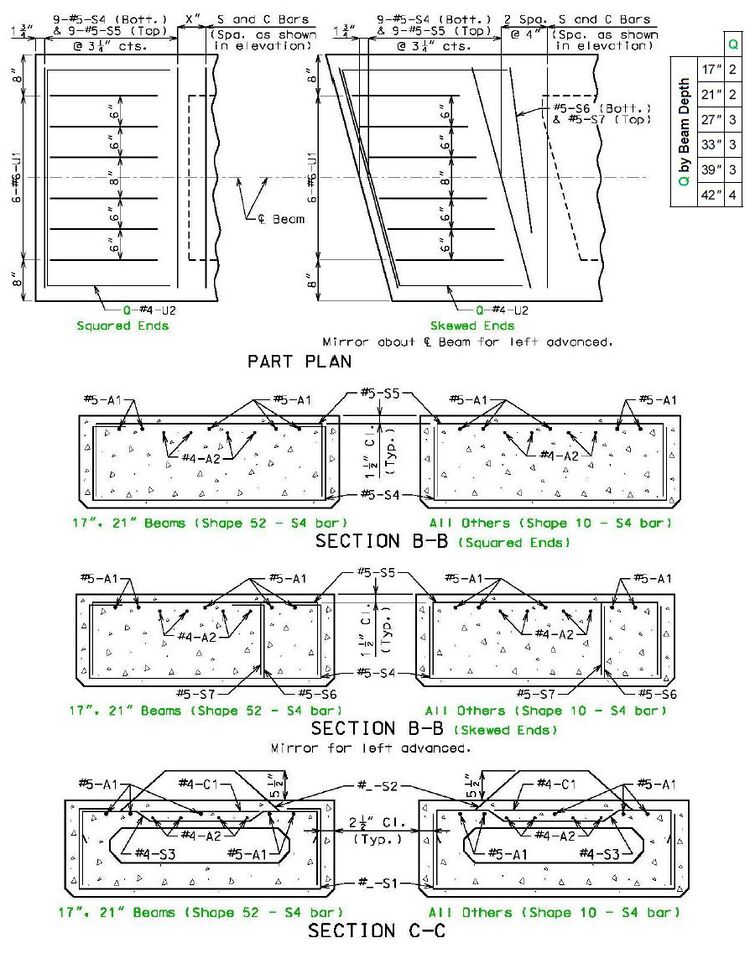
Bending Diagrams
Dimensions shown are out to out. Use symmetry for dimensions not shown. Use "ɑ" bars for squared-end beams. Use "b" bars for skewed-end beams.

![]() |
![]() |
For beams that have excessive haunch or beam steps, create new S2 bars and adjust heights in one-inch increments or provide #4 hairpin bars in accordance with EPG 751.10.1.14 Girder and Beam Haunch Reinforcement to ensure at least 2-inch embedment into slab.
751.21.3.6.3 Reinforcement
751.22.2.3 Flexure
Flexure capacity of girders shall be determined as the following.
Flexural resistance at strength limit state
Where:
= | Flexural resistance | |
= | Nominal flexural resistance | |
= | Total factored moment from Strength I load combination | |
= | Flexural resistance factor as calculated in LRFD 5.5.4.2 |
Negative moment reinforcement design
P/S I-girder shall be designed as a reinforced concrete section at regions of negative flexures (i.e., negative moments).
At least one-third of the total tensile reinforcement provided for negative moment at the support shall have an embedment length beyond the point of inflection not less than the specified development length of the bars used.
Slab longitudinal reinforcement that contributes to making the precast beam continuous over an intermediate bent shall be anchored in regions of the slab that can be shown to be crack-free at strength limit states. This reinforcement anchorage shall be staggered. Regular longitudinal slab reinforcement may be utilized as part of the total longitudinal reinforcement required.
Effective Slab Thickness
An effective slab thickness shall be used for design by deducting from the actual slab thickness a 1” integral, sacrificial wearing surface.
Design A1 reinforcement in the top flange
The A1 reinforcement shall resist the tensile force in a cracked section computed on the basis of an uncracked section.
For I girders and bulb-tee girders, A1 reinforcement shall consist of deformed bars (minimum #5 for Type 2, 3 and 4 and minimum #6 for Type 6, 7 and 8).
For NU girders, A1 reinforcement shall consist of the four 3/8-inch diameter reinforcement support strands with deformed bars added only as needed. The WWR in the top flange shall not be used for A1 reinforcement because there is insufficient clearance to splice the WWR.
See guidance on Bridge Standard Drawings (Prestressed I-Girders - PSI) for required lap lengths, if required.
Required steel area is equal to:
Where:
= , allowable tensile stress of mild steel, (ksi) | |
= Resultant of total tensile force computed on the basis of an uncracked section, (kips) |
Limits for reinforcement
The following criteria shall be considered only at composite stage.
Minimum amount of prestressed and non-prestressed tensile reinforcement shall be so that the factored flexural resistance, Mr, is at least equal to the lesser of:
- 1) Mcr LRFD Eq. 5.6.3.3-1
- 2) 1.33Mu
Where:
Mcr | = | Cracking moment, (kip-in.) |
Mu | = | Total factored moment from Strength I load combination, (kip-in.) |
751.22.3.7.2 Reinforcement
The reinforcement shall be detailed on the plan sheets for closed concrete intermediate diaphragms as shown below except:
- Bar marks revised as required.
- Abbreviations used as required.
- Add "(Typ.)" to dimensions and leader notes as appropriate.
All U bar reinforcement shall use stirrup bends.
All reinforcement in diaphragms shall be epoxy coated, except coating of dowel bars shall match the coating of intermediate bent reinforcement.
Coil ties and rods shall also be shown in the section near the diaphragm and the horizontal section near the top of diaphragm in accordance with EPG 751.22.3.10 Coil Inserts and Tie Rods.
Unless specified the details shown are for the same girder heights within a continuous girder series.
I Girders Type 2, 3, 4 and 6
Bulb-Tee Girders Type 7 and 8
![]() |
![]() | |
SECTION NEAR DIAPHRAGM (Normal to centerline of girders) |
||
![]() | ||
SECTION A-A (Skewed over 25°) | ||
![]() |
![]() |
Detailing Guidance: Green items are guidance only and shall not be shown on the plans. See Section A-A for I girders type 2, 3, 4 and 6 for differences in strand tie bars for bents skewed up to 25°. Bars will need to clear any shear blocks required for expansion bents. (ɑ) X equals layers of bent up strands (omit quantity if one layer). (b) 19 inches minimum for #4 bars and full available width for #6 bars. (c) 11" may be used if required for spacing. |
ELEVATION C-C | SECTION B-B (Normal) (Fixed Bent) |
NU Girders
NU 53 girders are shown in the following details. The details for other NU girder types are similar.
![]() |
![]() | ||||||||||||||||||||||||
SECTION NEAR DIAPHRAGM (Normal to centerline of girders) |
|||||||||||||||||||||||||
![]() | |||||||||||||||||||||||||
SECTION A-A (Skewed over 25°) | |||||||||||||||||||||||||
![]() |
![]() |
| |||||||||||||||||||||||
ELEVATION C-C | SECTION B-B (Normal) Fixed Bent | ||||||||||||||||||||||||
Detailing Guidance: Green items are guidance only and shall not be shown on the plans. See Section A-A for I girders type 2, 3, 4 and 6 for differences in strand tie bars for bents skewed up to 25°. Bars will need to clear any shear blocks required for expansion bents. (ɑ) X equals layers of bent up strands (omit quantity if one layer). (b) 19 inches minimum for #4 bars and full available width for #6 bars. |
Change in Girder Height
The following details are based on I Girders Type 2, 3, 4 and 6. The details are appropriate for bulb-tee and NU girders by substituting the appropriate reinforcing details from above. The reinforcement is that of the taller girder with additional #6 bars located under the shorter girder. The section near the diaphragm shall be from the perspective of the shorter girders. The differences from uniform girder height details are highlighted.
![]() |
![]() | ||||||||||||||||||
SECTION NEAR DIAPHRAGM (e) (Looking back station) (Normal to centerline of girders) |
|||||||||||||||||||
![]() | |||||||||||||||||||
SECTION A-A (Skewed over 25°) | |||||||||||||||||||
![]() |
Detailing Guidance: Green items are guidance only and shall not be shown on the plans. See Section A-A for I girders type 2, 3, 4 and 6 for differences in strand tie bars for bents skewed up to 25°. Bars will need to clear any shear blocks required for expansion bents. (ɑ) X equals layers of bent up strands (omit quantity if one layer). (b) 19 inches minimum for #4 bars and full available width for #6 bars. (c) Subtract one row for Type 2 and 3. Add one row for Type 6. (d) For squared bents replace H26 with H25. (e) Only required if shorter girders are down station from taller girders. |
| |||||||||||||||||
ELEVATION B-B (Normal) (Fixed Bent) Change in girder heights not allowed at expansion bents. |
751.22.3.8.2 Reinforcement
The reinforcement shall be detailed on the plan sheets for open concrete intermediate diaphragms as shown below except:
- Bar marks revised as required.
- Abbreviations used as required.
- Add "(Typ.)" to dimensions and leader notes as appropriate.
All U bar reinforcement shall use stirrup bends.
All reinforcement in diaphragms shall be epoxy coated.
Coil ties and rods shall also be shown in the section near the diaphragm and the horizontal section near the top of diaphragm in accordance with EPG 751.22.3.10 Coil Inserts and Tie Rods.
I Girders Type 2, 3, 4 and 6
![]() | |||||||||||||||||||||||||
SECTION NEAR DIAPHRAGM (Normal to centerline of girders) | |||||||||||||||||||||||||
![]() | |||||||||||||||||||||||||
SECTION A-A | |||||||||||||||||||||||||
![]() |
![]() |
| |||||||||||||||||||||||
SECTION B-B (Normal) |
|||||||||||||||||||||||||
Detailing Guidance: Green items are guidance only and shall not be shown on the plans. U21 are varied bars. (ɑ) Hook ends if length of bar is less than 88" (ℓd = 44"). (b) For squared bents replace with pairs of U23 bars. (c) X equals layers of bent up strands (omit quantity if one layer). (d) 19 inches minimum for #4 bars and full available width for #6 bars. |
Bulb-Tee Girders Type 7 and 8
![]() |
![]() | |
SECTION NEAR DIAPHRAGM (Normal to centerline of girders) |
||
![]() | ||
SECTION A-A | ||
![]() |
![]() |
Detailing Guidance: Green items are guidance only and shall not be shown on the plans. U31, U35, U36 & U37 are varied bars. (ɑ) Hook ends if length of bar is less than 88" (ℓd = 44"). (b) Replace with pairs of U36 bars for squared bents. (c) X equals layers of bent up strands (omit quantity if one layer). (d) 19 inches minimum for #4 bars and full available width for #6 bars. |
ELEVATION C-C | SECTION B-B (Normal) |
NU Girders
NU 53 girders are shown in the following details. The details for other NU girder types are similar.
![]() |
![]() | ||||||||||||||||||||||||||||||||||||||||
SECTION NEAR DIAPHRAGM (Normal to centerline of girders) |
|||||||||||||||||||||||||||||||||||||||||
![]() | |||||||||||||||||||||||||||||||||||||||||
SECTION A-A | |||||||||||||||||||||||||||||||||||||||||
![]() |
![]() |
| |||||||||||||||||||||||||||||||||||||||
ELEVATION C-C | SECTION B-B (Normal) |
||||||||||||||||||||||||||||||||||||||||
Detailing Guidance: Green items are guidance only and shall not be shown on the plans. U41, U44, U46 & U47 are varied bars. (ɑ) Hook ends if length of bar is less than 88" (ℓd = 44"). (b) For squared bents replace with pairs of U23 bars. (c) X equals layers of bent up strands (omit quantity if one layer). (d) 19 inches minimum for #4 bars and full available width for #6 bars. (e) NU 78 requires another row. |
751.22.3.9.2 Reinforcement
The reinforcement shall be detailed on the plan sheets for concrete end diaphragms as shown below except:
- Bar marks revised as required.
- Abbreviations used as required.
- Add "(Typ.)" to dimensions and leader notes as appropriate.
All U bar reinforcement shall use stirrup bends.
All reinforcement in diaphragms shall be epoxy coated.
Coil ties and rods shall also be shown in the section near the diaphragm and the horizontal section near the top of diaphragm in accordance with EPG 751.22.3.10 Coil Inserts and Tie Rods.
I Girders Type 2, 3, 4 and 6
![]() | |||||||||||||||||||||||||
SECTION NEAR DIAPHRAGM (Normal to centerline of girders) | |||||||||||||||||||||||||
![]() | |||||||||||||||||||||||||
SECTION A-A | |||||||||||||||||||||||||
![]() |
![]() |
![]() | |||||||||||||||||||||||
Small Expansion Device (End Bend with Sliding Slab Similar) |
Finger Plate Expansion Device | ||||||||||||||||||||||||
SECTION B-B (Normal) | |||||||||||||||||||||||||
Detailing Guidance: Green items are guidance only and shall not be shown on the plans. Use full available width for lap of all stirrup bars except the upper legs at a finger plate expansion device are developed as specified. U21 and U27 are varied bars. (ɑ) Hook ends of H200 bars if length is less than 66" (ℓd = 33"). (b) For squared bents replace both with Pr.-#4-U23. (c) For squared bents replace U24 with U22. (d) For finger plate expansion devices replace with #4-U20 & #4-U26. (e) For finger plate expansion devices replace with #6-U21 & #6-U27. |
|
Bulb-Tee Girders Type 7 and 8
![]() | ||
SECTION NEAR DIAPHRAGM (Normal to centerline of girders) | ||
![]() | ||
SECTION A-A | ||
![]() |
![]() | |
Detailing Guidance: Green items are guidance only and shall not be shown on the plans. See Section B-B for I Girders for differences due to a finger plate expansion device. Lap #4 stirrup bars 19 inches if available otherwise lap all stirrup bars full available width. H301, U301, U304, U306, U308 and U310 are varied bars. (ɑ) Hook ends of H300 bars if length is less than 66" (ℓd = 33"). (b) For squared bents replace both with Pr.-#4-U306. (c) For squared bents replace U307 with U303, U308 with U304 and U309 with U305. | ||
SECTION B-B (Normal) |
NU Girders
NU 53 girders are shown in the following details. The details for other NU girder types are similar.
![]() | |||||||||||||||||||||||||||||||||||||||||
SECTION NEAR DIAPHRAGM (Normal to centerline of girders) | |||||||||||||||||||||||||||||||||||||||||
![]() | |||||||||||||||||||||||||||||||||||||||||
SECTION A-A | |||||||||||||||||||||||||||||||||||||||||
![]() |
![]() |
| |||||||||||||||||||||||||||||||||||||||
SECTION B-B (Normal) |
|||||||||||||||||||||||||||||||||||||||||
Detailing Guidance: Green items are guidance only and shall not be shown on the plans. See Section B-B for I Girders for differences due to a finger plate expansion device. Lap #4 stirrup bars 19 inches if available otherwise lap all stirrup bars full available width. H401, U401, U404, U406, U408 and U410 are varied bars. Do not vary U403 and U407 bars. Horizontal legs are controlled by the minimum allowable space on each end of diaphragm. (ɑ) Hook ends of H400 bars if length is less than 66" (ℓd = 33"). (b) For squared bents replace both with 2 Pr.-#4-U406. (c) For squared bents replace U407 with U403, U408 with U404 and U409 with U405. (d) NU 78 requires another row of H401 and H402 (4-#6-H401 & 3-#6-H402 in Section A-A). |
751.22.3.9.3 Closed Diaphragm
Use only when expansion device connects prestressed girder series and steel girder series, and laminated neoprene pads are used under the prestressed girders in accordance with expansion limits of these bearings and only with the approval of the Structural Project Manager or Structural Liaison Engineer.
The simplified detail below is for I girders. The actual details required on the plans can be developed for all girder types by substituting the dimensions and reinforcement of the corresponding section near the diaphragm detail of EPG 751.22.3.7 and the dimensions from the corresponding Section A-A of EPG 751.22.3.9.1 Dimensions.
![]() |
![]() |
SECTION THRU CLOSED END DIAPHRAGM |
751.31.3.1 Beam Cap
![]() |
![]() |
![]() | |
PART ELEVATION | SECTION A-A | SECTION B-B | |
(Single Stirrups) | |||
![]() |
![]() |
![]() | |
PART PLAN | SECTION A-A | SECTION B-B | |
(Double Stirrups) | |||
![]() |
![]() | ||
TRANSVERSE BEAM STEPS OVER 3 INCHES (Also, steps accumulating over 3 inches) |
SLOPED BEAMS (Steps over 3 inches on high side) | ||
![]() |
![]() |
(1) #4 @ 12” cts. (Min.) See EPG 751.5.9.2.8 for development and lap splice lengths not given or lengths for See EPG 751.5.9.2.2 for epoxy coated reinforcement requirements. See EPG 751.13.1.4 for details of protective coating and sloping top of beam to drain | |
Over 3 Inches Thru 12 Inches | Over 12 Inches | ||
LONGITUDINAL BEAM STEPS | |||
![]() | |||
REINFORCEMENT UNDER BEARINGS |
751.31.3.2 Column
![]() |
![]() | |
MINIMUM SPACING AT LAP SPLICES | ALTERNATE DOWEL PLACEMENT | |
* | Use alternate detail only with approval of Structural Project Manager and then design column reinforcement using the smaller ring diameter. | |
A = | 4 1/2” minimum spacing center-to-center. | |
B = | 2” clear spacing for bar sizes thru #10. | |
2 1/2” clear spacing for bar sizes #11 and #14. | ||
3 1/2” clear spacing for bar size #18. | ||
![]() | ||
STIRRUP LAP DETAIL AND STAGGER NOTE | ||
Lap splices for closed circular ties shall be provided and staggered in accordance with LRFD 5.10.6.3. | ||
Lap length of 1.3 ld (or Class B) for closed stirrup/ties shall be provided in accordance with LRFD 5.11.2.6.4. | ||
Lap length for #4 stirrup bars (4” min. spacing, f’c = 3 ksi, and clear cover = 1½”) equals 24” for uncoated bars and 28” for epoxy coated bars. | ||
For lap length for other scenarios, see EPG 751.5.9.2.8 Development and Lap Splices. |
Column Diameter | Minimum Reinforcement2,3 | Minimum Lap Splice | |
---|---|---|---|
Uncoated (f’c = 3ksi) (Cl. = 1½”) |
Epoxy Coated (f’c = 3ksi) (Cl. = 1½”) | ||
3’-0” | By Design | NA | NA |
3’-6” | By Design | NA | NA |
4’-0” | #6 @ 5” | 47” | 61” |
4’-6” | #5 @ 5” | 34” | 44” |
5’-0” | #4 @ 5” | 24” | 28” |
5’-6” | #4 @ 10” | 24” | 28” |
6’-0” | #4 @ 12” | 24” | 28” |
1 See EPG 751.2.2.6 Other Loads to determine if a pier requires design for collision loads.
3 The shear reinforcement tabulated is adequate for collision but may not be adequate for other design |
- Columns shall be reinforced using stirrup ties, unless excessive reinforcement is required, in which case spirals shall be used.
- Show spiral details of Fig. 751.9.3.1.7.4 on the bridge plans if spirals are used for bridge in non-seismic area. Anchorage of spiral reinforcement shall be provided by 1 ½ extra turns of spiral reinforcement at each end of the spiral unit.
![]() |
(1) Location 2 development length. (2) Check clearance to concrete piles. See EPG 751.5.9.2.8 for development and lap splice lengths not given or lengths for scenarios other than those shown. Provide standard hooks if required. See EPG 751.5.9.2.2 for epoxy coated reinforcement requirements. |
751.31.3.3 Web Beam
![]() |
![]() | |
PART ELEVATION | SECTION THRU WEB BEAM | |
![]() |
(1) L/4 + d, but not less than theoretical cut-off + development length.
(2) Location 2 development length, 4” to 12” spacing, 1½” clear, f'c = 3 ksi:
(3) Location 1 development length, f'c = 3 ksi:
(4) Location 2 development length. (5) Location 2 Class B lap splice. (6) Maximum spacing shall be 6" or 1/5 development length for noncontact lap splice. See EPG 751.5.9.2.8 for development and lap splice lengths See EPG 751.5.9.2.2 for epoxy coated reinforcement requirements. | |
VARIABLE DIAMETER COLUMN (Without Seismic Detailing) |
751.31.3.4 Tie Beam
![]() |
![]() | |
CONSTANT COLUMN DIAMETER | CHANGE IN COLUMN DIAMETER | |
PART ELEVATION AT TIE BEAM AND COLUMN | ||
![]() |
(1) Location 1 development length, f'c = 3 ksi: See EPG 751.5.9.2.8 for development and lap splice lengths not given or for lengths See EPG 751.5.9.2.2 for epoxy coated reinforcement requirements. | |
SECTION THRU TIE BEAM |
751.31.3.5 Hammer Head Type
![]() |
![]() |
PART PLAN | SECTION A-A |
![]() |
![]() |
PART ELEVATION | SECTION B-B |
(1) #4 @ 12” cts. (Min.)
(2) Hook bars if cantilever is less than the required Location 1 development length. (3) Location 2 development length. (4) Location 2 Class B lap splice, f'c = 3 ksi:
(5) Location 1 Class B lap splice, f'c = 3 ksi:
(6) See EPG 751.2.2.6 and 751.31.2.2 for collision requirements. (7) See LRFD 5.10.6.3 for tie requirements. See EPG 751.5.9.2.8 for development and lap splice lengths not given or for lengths See EPG 751.5.9.2.2 for epoxy coated reinforcement requirements. |
751.32.4.1 Typical Pile Cap Bent
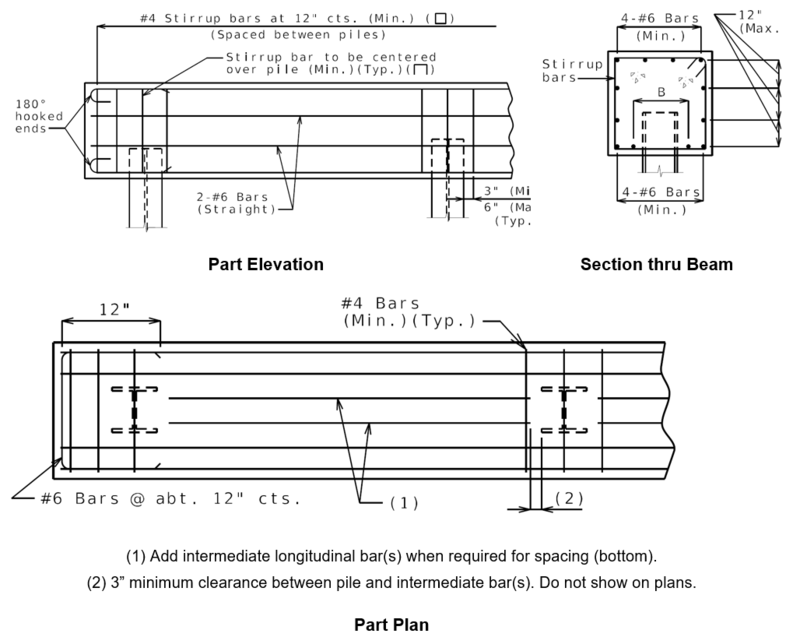
Note: | Locate #4 bars "![]() ![]() |
For epoxy coated reinforcement requirements, see EPG 751.5.9.2.2 Epoxy Coated Reinforcement Requirements. Details of protective coating and sloping top of beam to drain shall be used when below an expansion device. | |
When dimension “B” is required to be greater than 15” to clear piles by 1 ½”, typical when HP14 and CIP14 or larger diameter piles are used, add intermediate longitudinal bar(s) between piles. The spacing between intermediate bar(s) and full length bars shall not be greater than required by crack control provisions. Ends of intermediate bar(s) shall be hooked. |
![]() |
![]() |
(1) 6” (Max.), add #6 bars as needed.
See EPG 751.5.9.2.8 for development and lap splice lengths not given or |
751.35.4.1 Wide Flange Beams & Plate Girders
![]() | ||||
Part Section Near End Bent | ||||
![]() |
![]() | |||
Section A-A | Section B-B | Section C-C | Section D-D | |
![]() |
![]() | |||
Alternate Section A-A | Alternate Section B-B | Alternate Section C-C | Alternate Section D-D | |
Detailing Guidance: | ||||
Green items are guidance only and shall not be shown plans. | ||||
Place all U bars and V pairs parallel to centerline roadway. | ||||
Keep 1 1/2" clearance between the piles and the U1 or U2 bars. | ||||
Keep 1 1/2" clearance between the beams or girders and the U1 or V1 bars. | ||||
Keep 1 1/2" clearance between the angles of girder chairs and the U2 or U3 bars. | ||||
Replace U1 bars with U3 bars at piles under beams or girders. | ||||
Replace U1 bars with V1 bars at piles between beams or girders. | ||||
When dimension “A” is required to be greater than 15” to clear piles by 1 ½”, typical when HP14 and CIP14 or larger diameter piles are used, add intermediate longitudinal bar(s) between piles. The spacing between intermediate bar(s) and full length bars shall not be greater than required by crack control provisions. Ends of intermediate bar(s) shall be hooked. | ||||
See EPG 751.50 G1 Concrete Bents for appropriate notes to be placed with details. | ||||
(1) #6-U bar (![]() | ||||
(2) U4 bars (![]() ![]() ![]() stirrup hooks may be required for U4 bars ( ![]() minus 1” clear. | ||||
(3) U1 bars (![]() lap length requirement across length of diaphragm. For shallow beams stirrup hooks may be required for U1 bars ( ![]() bars ( ![]() | ||||
(4) Stirrups shall clear step by 1 1/2" minimum, if not lengthen step or skew step. | ||||
(5) #6-V bars at no more than 9” centers at the end of girders or beams. | ||||
(6) #5-U bars (15”H x 24”V) @ about 12" centers placed parallel to centerline roadway. When approach slab haunch is expected to be greater than 18” at the roadway crown at the end of slab, slope the approach slab notch providing 12” of constant approach slab haunch or with SPM or SLE approval greater than 18” approach slab haunch may be used but increase vertical leg length of #5-U bars to ensure 12” minimum embedment. For shallow beams where 12” embedment is not available adjust length of vertical leg and extend to top of beam minus 1” clear. | ||||
(7) With SPM or SLE approval a 24” splice may be used in combination with specifying 2” cover to U bars and V bars if doing so avoids the need for using hooked bars. | ||||
(8) See tables for 1 1/16" round hole spacing for #6-H bars. | ||||
(9) Same number of bars as 1 1/16" round holes in beam or girder. | ||||
(10) Add intermediate longitudinal bar(s) when required for spacing. Keep 3” minimum clearance between the pile and intermediate longitudinal bar(s). |
751.35.4.2 Prestressed I-Girders, Bulb-Tee Girders and NU-Girders
![]() | ||||
Part Section Near End Bent | ||||
![]() |
![]() | |||
Section A-A | Section B-B | Section C-C | Section D-D | |
![]() |
![]() | |||
Alternate Section A-A | Alternate Section B-B | Alternate Section C-C | Alternate Section D-D | |
Detailing Guidance: | ||||
Green items are guidance only and shall not be shown plans. | ||||
Place all U bars and V pairs parallel to centerline roadway. | ||||
Keep 1 1/2" clearance between the piles and the U1 or U2 bars. | ||||
Keep 1 1/2" clearance between the beams or girders and the U1 or V1 bars. | ||||
Keep 1 1/2" clearance between the angles of girder chairs and the U2 or U3 bars. | ||||
Replace U1 bars with U3 bars at piles under beams or girders. | ||||
Replace U1 bars with V1 bars at piles between beams or girders. | ||||
When dimension “A” is required to be greater than 15” to clear piles by 1 ½”, typical when HP14 and CIP14 or larger diameter piles are used, add intermediate longitudinal bar(s) between piles. The spacing between intermediate bar(s) and full length bars shall not be greater than required by crack control provisions. Ends of intermediate bar(s) shall be hooked. | ||||
See EPG 751.50 G1 Concrete Bents for appropriate notes to be placed with details. | ||||
(1) #6-U bar (![]() | ||||
(2) U4 bars (![]() ![]() ![]() stirrup hooks may be required for U4 bars ( ![]() minus 1” clear. | ||||
(3) U1 bars (![]() lap length requirement across length of diaphragm. For shallow beams stirrup hooks may be required for U1 bars ( ![]() bars ( ![]() | ||||
(4) Stirrups shall clear step by 1 1/2" minimum, if not lengthen step or skew step. | ||||
(5) #6-V bars at no more than 9” centers at the end of girders or beams. | ||||
(6) #5-U bars (15”H x 24”V) @ about 12" centers placed parallel to centerline roadway. When approach slab haunch is expected to be greater than 18” at the roadway crown at the end of slab, slope the approach slab notch providing 12” of constant approach slab haunch or with SPM or SLE approval greater than 18” approach slab haunch may be used but increase vertical leg length of #5-U bars to ensure 12” minimum embedment. For shallow beams where 12” embedment is not available adjust length of vertical leg and extend to top of beam minus 1” clear. | ||||
(7) With SPM or SLE approval a 24” splice may be used in combination with specifying 2” cover to U bars and V bars if doing so avoids the need for using hooked bars. | ||||
(8) Add intermediate longitudinal bar(s) when required for spacing. Keep 3” minimum clearance between the pile and intermediate longitudinal bar(s). |
751.35.4.3 Wing Reinforcement
![]() |
![]() |
Elevation Of Wing | Typical Section Thru Wing |
![]() | |
Part Plan (Squared) | |
![]() | |
Part Plan (Skewed) | |
Detailing Guidance: | |
Green items are guidance only and shall not be shown plans. | |
Bar marks shown are for these details only. Vary as needed. | |
K bars not shown in the Elevation of Wing for clarity. For details of K bars, see EPG 751.12.1.4.3 End of Barrier Reinforcement for Type B barrier and EPG 751.12.1.3.3 End of Barrier Reinforcement for Type D and H barriers. | |
See EPG 751.35.3.3 for chamfer detail. | |
(a) Use dimension that provides a minimum of 3" center to center spacing between #6 bars placed horizontally and #8 bars placed with grade. See SPM or SLE if spacing at one end exceeds 8 inches due to grade. | |
(b) Use construction joint on steel structures only. | |
(c) 6 3/8” min and 11 3/8” max. If unable to get dimension to fall within this range using 8-inch centers, then use 6 3/8” and use “@ abt. 8” cts.” | |
(d) Use 66.5” for obtuse corner of bents skewed 55 degrees or greater. | |
(e) Use 54.5” for obtuse corner of bents skewed 55 degrees or greater. | |
(f) See 751.50_Standard_Detailing_Notes#G1._Concrete_Bents EPG 751.50 G1 Concrete Bents for note (G1.7) required for the #6-F bars. | |
(g) Use 90 degree standard hook in seismic areas. | |
(h) Adjust as needed if girder web prevents proper placement of bars (i.e., if ALL bars would need to be bent in field according to note G1.7). Rotate leg 90° for box beams. If necessary, the leg may be rotated 90° for other prestressed girder shapes. |
H10a. Cast-In-Place Permanent Barrier
The following notes shall be placed in the General Notes on the elevation sheet.
(H10.0.1) Use note if slip forming is allowed. Add asterisk to all C-bar leader notes and the one fiberglass bar leader note in the elevation of barrier.
- * Slip-formed option only.
(H10.0.2) Both methods may be used unless otherwise specified on Bridge Memorandum.
- Conventional forming or slip forming may shall be used. Saw cut joints may be used with conventional forming.
(H10.1) Exclude underlined part for single span bridges.
- Top of barrier shall be built parallel to grade with barrier joints (except at end bents) normal to grade.
(H10.2)
- All exposed edges of barrier shall have either a 1/2-inch radius or a 3/8-inch bevel, unless otherwise noted.
(H10.3)
- Payment for all concrete and reinforcement, complete in place, will be considered completely covered by the contract unit price for Type A B C D H Barrier per linear foot.
(H10.4)
- Concrete in barrier shall be Class B-1.
(H10.5) Use for Type B, D or H barrier. Include “left” or ”right” and exclude “for each structure” when barriers on each side of the bridge are not the same type.
- Measurement of barrier is to the nearest linear foot for each structure, measured along the left right outside top of slab from end of wing to end of wing slab to end of slab.
(H10.7) Use for Type A or C barriers.
- Measurement of barrier is to the nearest linear foot, measured along the top of slab at centerline median from end of bridge approach slab to end of bridge approach slab.
(H10.7.1) Use for all barriers (see Barrier Wall Delineation).
- Concrete traffic barrier delineators shall be placed on top of the barrier as shown on Missouri Standard Plans 617.10 and in accordance with Sec 617. Delineators on bridges with two-lane, two-way traffic shall have retroreflective sheeting on both sides. Concrete traffic barrier delineators will be considered completely covered by the contract unit price for Type A B C D H Barrier.
Below is additional guidance for using Note H10.7.1: |
Bridges with two-lane, two-way traffic shall have retroreflective sheeting on both sides of the delineators. For two-lane, one-way traffic, retroreflective sheeting may be on one side only unless crossroad or entranceway traffic is just beyond exit to bridge and wrong way driving is to be discouraged with retroreflective sheeting on both sides of the delineators, (white and red in this case). "Delineators on bridges with two-lane, two-way traffic shall have retroreflective sheeting on both sides" may be modified, as required. For Type A and C barriers, retroreflective sheeting should be used on both sides of the delineators where there is not more than four lanes divided. |
On bridges with more than two lanes, retroreflective sheeting is not required on both sides of the delineators. The perception of a narrowing roadway at the bridge is of lesser consequence in terms of requiring guidance devices and does not warrant retroreflective sheeting on both sides of the delineators. "Delineators on bridges with two-lane, two-way traffic shall have retroreflective sheeting on both sides" may be removed at the discretion of the design team. |
(H10.7.2)
- Joint sealant and backer rods shall be in accordance with Sec 717 for silicone joint sealant for saw cut and formed joints.
(H10.7.3) Use note if slip forming is allowed.
- For slip-formed option, both sides of barrier shall have a vertically broomed finish and the top shall have a transversely broomed finish.
(H10.7.4) Use for all grade separations except over railroads and county roads.
- Plastic waterstop shall not be used with saw cut joints.
The following three notes shall be placed under section thru barrier.
(H10.8)
- Use a minimum lap of 2'-6" for #5 horizontal barrier bars.
(H10.9) Areas shown are for standard barrier heights and a two percent cross slope.
- The cross-sectional area above the slab is * square feet.
* 2.98 for a Type A barrier. 2.27 for a Type B barrier. 4.69 for a Type C barrier. 3.52 for a Type D barrier. 3.59 for a Type D barrier used as a median. 2.89 for a Type H barrier
(H10.9.1) Add (2) to the dimension for the top of slab to top of the R2 bar.
- (2) To top of bar
The following three notes shall be used for double-tee structures.
(H10.10)
- Coil inserts shall have a concrete ultimate pullout strength of not less than 36,000 pounds in 5000 psi concrete and an ultimate tensile strength of not less than 36,000 pounds.
(H10.11)
- Threaded coil rods shall have an ultimate capacity of 36,000 pounds. All coil inserts and threaded coil rods shall be galvanized in accordance with AASHTO M 232 (ASTM A153), Class C.
(H10.12)
- Payment for furnishing and installing coil inserts and threaded coil rods will be considered completely covered by the contract unit price for Type A B C D H Barrier.
The following two notes, when appropriate, shall be placed under the title of the elevation of barrier.
(H10.12.1) Dimensions shall be horizontal unless otherwise specified on Bridge Memorandum.
- Longitudinal dimensions are horizontal arc dimensions.
(H10.12.2)
- Longitudinal dimensions are along top of barrier outside edge of slab parallel to grade.
The following two notes shall be placed under the permissible alternate bar shape detail.
(H10.13) Use R2 for Type D or H barriers, R3 for Type B barrier and M2 for two separate Type D barriers used as a median. Add (4) to the combined #5 bar leader note. Exclude note and associated detail for CIP slabs.
- (4) The R2 R3 M2 bar and #5 bottom transverse slab bar in cantilever (prestressed panels only) combination may be furnished as one bar as shown, at the contractor's option.
(H10.14) Use R1 for Type B, D or H barriers. Use M1 for two separate Type D barriers used as a median. Add (3) to the two separated #5 bar leader notes.
- (3) The R1 M1 bar may be separated into two bars as shown, at the contractor's option, only when slip forming is not used. (All dimensions are out to out.)
(H10.15) Use note if slip forming is allowed. Place under the part elevation of barrier and add (1) to fiberglass bar leader notes in the section thru saw cut joint and part elevation of barrier.
- (1) Four feet long, centered on joint, slip-formed option only
Place general notes H10.19, H10.20 and H10.7.1 on the barrier at end bents sheet with notes H10.19 and H10.20 under the Reinforcing Steel heading.
(H10.19)
- Minimum clearance to reinforcing steel shall be 1 1/2" except as shown for bars embedded into end bent.
(H10.20) Use for Type B barrier only. Use 2’-4” and K10 bars for barrier ending on wing walls adding K13 bars with two different wing lengths. Will need to add more bars if more than two different wing lengths exist. Use 2’-6” and R6 bars for barrier ending on bridge deck.
- Use a minimum lap of 2'-4" 2’-6” between K9 and K10 or K13 R6 bars.
(H10.21) Place note under the K Bar Permissible Alternate Shape detail on the barrier at end bents sheet. Use K1 and K2 for Type B barrier; K9 and K10 for Type D barrier; K3 and K5 for Type H barrier.
- The K1 and K2 K9 and K10 K3 and K5 bar combination may be furnished as one bar as shown, at the contractor's option.
K1. General
(K1.1) Use for Bridge Approach Slab (Major Road) and omit underlined part for concrete sub-class Bridge Approach Slab (Minor Road).
- All concrete for the bridge approach slab and sleeper slab shall be in accordance with Sec 503 (f'c = 4,000 psi).
(K1.2)
- All joint filler shall be in accordance with Sec 1057 for preformed fiber expansion joint filler, except as noted.
(K1.3) Use for Bridge Approach Slab (Major Road) and omit underlined part for concrete sub-class Bridge Approach Slab (Minor Road).
- The reinforcing steel in the bridge approach slab and the sleeper slab shall be epoxy coated Grade 60 with Fy = 60,000 psi.
(K1.4)
- Minimum clearance to reinforcing steel shall be 1 1/2", unless otherwise shown.
(K1.5.1) Use for Bridge Approach Slab (Major Road).
- The reinforcing steel in the bridge approach slab and the sleeper slab shall be continuous. The transverse reinforcing steel may be made continuous by providing a minimum lap splice of 24 inches for #5 bars and 40 inches for #6 bars, or by mechanical bar splice.
(K1.5.2) Use for Bridge Approach Slab (Minor Road).
- The reinforcing steel in the bridge approach slab shall be continuous. The transverse reinforcing steel may be made continuous by providing a minimum lap splice of 26 inches for #4 bars, or by mechanical bar splice.
(K1.6) Use underline portion when mechanical bar splices are required due to staged construction.
- Mechanical bar splices shall be in accordance with Sec 710. (Estimated ____ splices per slab)
(K1.7)
- Seal joint between vertical face of approach slab and wing with sealant in accordance with Sec 717 for silicone joint sealant for saw cut and formed joints.
(K1.11)
- The contractor shall pour and satisfactorily finish the bridge semi-deep slab before placing the bridge approach slab.
(K1.12)
- Longitudinal construction joints in approach slab and sleeper slab shall be aligned with longitudinal construction joints in bridge semi-deep slab.
(K1.13) Use for Bridge Approach Slab (Major Road)
- Payment for furnishing all materials, labor and excavation necessary to construct the approach slab, including the timber header, sleeper slab, underdrain, Type 5 aggregate base, joint filler and all other appurtenances and incidental work as shown on this sheet, complete in place, will be considered completely covered by the contract unit price for Bridge Approach Slab (Major Road) per square yard.
(K1.14a) Use for Bridge Approach Slab (Minor) – Concrete Slab Only
- Payment for furnishing all materials, labor and excavation necessary to construct the concrete bridge approach slab, including the timber header, underdrain, Type 5 aggregate base, joint filler and all other appurtenances and incidental work as shown on this sheet, complete in place, will be considered completely covered by the contract unit price for Bridge Approach Slab (Minor) per square yard.
(K1.14b) Use for Bridge Approach Slab (Minor) – Asphalt Slab Only
- Payment for furnishing all materials, labor and excavation necessary to construct the asphalt bridge approach slab, including tack, curb and Type 5 aggregate base within the pay limits shown, complete in place, will be considered completely covered by the contract unit price for Bridge Approach Slab (Minor) per square yard.
(K1.15) Use for Bridge Approach Slab (Major Road) and Bridge Approach Slab (Minor Road) – Concrete Slab Only
- For concrete approach pavement details, see roadway plans.
(K1.16) Use for Bridge Approach Slab (Major Road)
- See Missouri Standard Plan 609.00 for details of Type A curb.
(K1.17) Use for Bridge Approach Slab (Minor Road) – Asphalt Slab Only
- See Missouri Standard Plan 609.00 for details of Type S curb.
(K1.18)
- With the approval of the engineer, the contractor may crown the bottom of the approach slab to match the crown of the roadway surface.
(K1.19) [MS Cell] Use boxed note for Bridge Approach Slab (Minor Road)
MoDOT Construction personnel will indicate the bridge approach slab used for this structure: | |
□ Concrete Bridge Approach Slab | |
□ Asphalt Bridge Approach Slab |
(K1.20)
- Drain pipe may be either 6" diameter corrugated metallic-coated pipe underdrain, 4" diameter corrugated polyvinyl chloride (PVC) drain pipe, or 4" diameter corrugated polyethylene (PE) drain pipe.
REVISION REQUEST 4030
620.5.4 Delineator Placement and Spacing (MUTCD Section 3F.04)
Guidance. Delineators should be mounted on suitable supports at a mount height, measured vertically from the bottom of the lowest retroreflective device to the elevation of the near edge of the roadway, of approximately 4 ft., see Standard Plans 903.00.
Option. When mounted on the face of or on top of guardrails or other longitudinal barriers, delineators may be mounted at a lower elevation than the normal delineator height recommended in the preceding paragraph.
Delineators should be placed 2 to 8 ft. outside the outer edge of the shoulder, or if appropriate, in line with the roadside barrier that is 8 ft. or less outside the outer edge of the shoulder.
Delineators should be placed at a constant distance from the edge of the roadway, except that where an obstruction intrudes into the space between the pavement edge and the extension of the line of the delineators. The delineators should be transitioned to be in line with or inside the innermost edge of the obstruction. If the obstruction is a guardrail or other longitudinal barrier, the delineators should be transitioned to be just behind, directly above (in line with), or on the innermost edge of the guardrail or longitudinal barrier. Channel post mounted delineators should not be installed behind guardrail if guardrail delineation is present.
Delineators should be spaced 200 ft. to 530 ft. apart on mainline tangent sections. Delineators should be spaced 100 ft. apart on ramp tangent sections.
Support. Examples of delineator installations are shown in Fig. 620.5.4.1, Examples of Delineator Placement.
Option. When uniform spacing is interrupted by such features as driveways and intersections, delineators which would ordinarily be located within the features may be relocated in either direction for a distance not exceeding one quarter of the uniform spacing. Delineators still falling within such features may be eliminated.
Delineators may be transitioned in advance of a lane transition or obstruction as a guide for oncoming traffic.
Guidance. The spacing of delineators should be adjusted on approaches to and throughout horizontal curves so that several delineators are always simultaneously visible to the road user. The approximate spacing is shown in the Approximate Spacing for Delineators on Horizontal Curves Table.
Radius of Curve, R ft. | Approximate Spacing on Curve, S ft. |
---|---|
50 | 20 |
115 | 25 |
180 | 35 |
250 | 40 |
300 | 50 |
400 | 55 |
500 | 65 |
600 | 70 |
700 | 75 |
800 | 80 |
900 | 85 |
1,000 | 90 |
Distances were rounded to the nearest 5 ft. |
Spacing for specific radii may be interpolated from table. The minimum spacing should be 20 feet. The spacing on curves should not exceed 300 feet. In advance of or beyond a curve, and proceeding away from the end of the curve, the spacing of the first delineator is 2S, the second 3S, and the third 6S but not to exceed 300 feet. S refers to the delineator spacing for specific radii computed from the formula (Formula applies to measurements in feet only). |
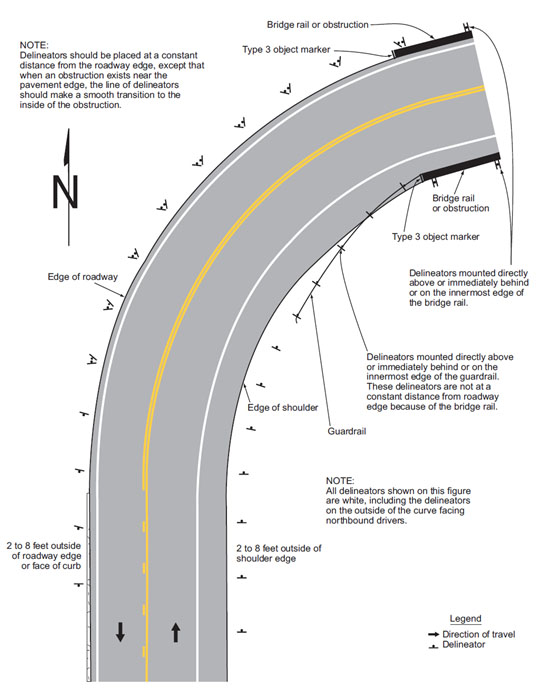
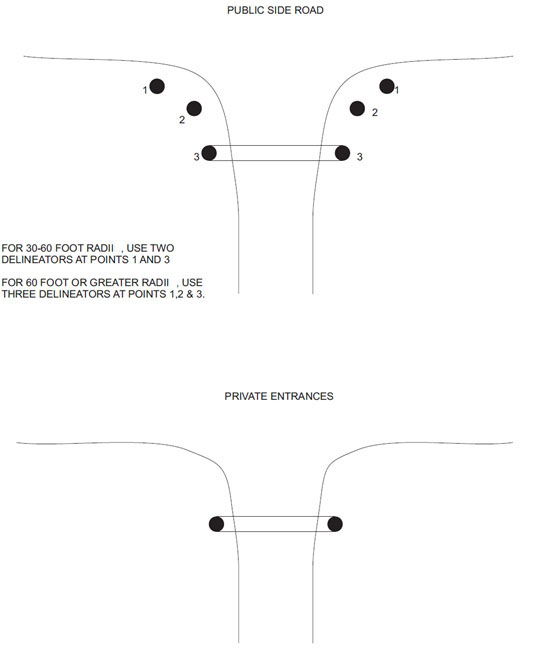
620.5.5 Guardrail Delineation
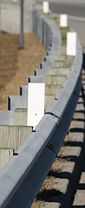
Standard. All guardrail shall be delineated in accordance with Section 606.10.2.3 of the Standard Specifications. The design of the guardrail delineators shall be in accordance with Standard Plans 903.00 and Standard Specification Sec 1065. The color of the retroreflective sheeting used shall match the color of the adjacent edgeline. If no edgeline is present, white shall be used on the right side facing approaching traffic.
On two-lane roads with two-way traffic the guardrail shall be delineated with white retroreflecitve sheeting on both sides of the delineator, including at bridge approaches.
Standard. If guardrail is present at off ramps, the back side of the guardrail delineator shall be red retroreflective sheeting. The red sheeting is used on the back side of guardrail delineators from the crossroad to the start of the deceleration lane on the main line.
Option. The use of the red sheeting on the back side of guardrail delineators may be used wherever there is a need to discourage wrong way driving.
620.5.6 Barrier Wall Delineation
Standard. Permanent barrier walls and bridge barrier walls shall be delineated in accordance with Section 617.30 of the Standard Specifications. The design of barrier wall delineators shall be in accordance with Standard Plan 903.00 and Standard Specification Sec 1065. The color of the barrier delineators shall match the color of the adjacent edgeline.
Guidance. Where there is traffic on both sides of a barrier wall, a two sided delineator with retroreflective sheeting on both sides, should be used. On two-lane roads the bridge barrier walls should be delineated with white retroreflective sheeting on both sides of the delineator.
Standard. If a barrier wall is present at off ramps, the back side of the barrier wall delineator shall be red retroreflective sheeting. The red sheeting is used on the back side of barrier wall delineators from the crossroad to the start of the deceleration lane on the main line.
Option. The use of the red sheeting on the back side of barrier wall delineators may be used wherever there is a need to discourage wrong way driving.
903.2.25.4 Quantity Computations
Standard. Signs and posts will each be paid for individually. This includes emergency reference markers and object markers. Combined unit prices for sign and support combinations have been discontinued. All signs including stop signs, object markers, emergency reference markers and signal signs shall be totaled on Form D-30 in four categories: Flat Sheet (FS), Flat Sheet Fluorescent (FSF), Structural (ST) and Structural Fluorescent. Structural signs’ width and height are designed to the nearest foot. Each standard, non-standard or special sign shall be calculated to the nearest 0.1 sq. ft., subtotaled to the nearest 0.1 sq. ft., and final pay total should be to the nearest 1.0 sq. ft.
All post quantities shall be calculated and totaled on Form D-29. All post lengths shall be calculated in increments of 0.25 ft. including the length that extends into the concrete footing or ground as shown on the standard plans. All U-channel post lengths shall include the full length of both pieces when overlaps are required. The post length for wide flange and pipe posts shall be multiplied by the pounds per foot (lb/ft) factor, as shown in the standard plans; each sign's posts are subtotaled to the nearest pound; all sign posts are subtotaled; and the final pay totals are shown to the nearest 10 pounds. All U-channel, wood and perforated square steel tube post length quantities shall be totaled and rounded to the nearest foot. For perforated square steel tube posts, an additional pay item shall be included for the anchor sleeve which is paid for by the linear foot for each post used (and may also include a soil plate). See the Post and Anchor Data Table in Standard Plan 903.03 to select the necessary anchor size. Omni-Directional anchors may be used for installation in weak or loose soil conditions.
Concrete for sign support structures shall be totaled on Form D-29. Concrete for overhead structure foundations shall be bolted down. Concrete for all post-mounted sign foundations shall be embedded. Bolted down and embedded quantities shall be calculated for each sign to the nearest 0.01 cubic yard, subtotaled to the nearest 0.01 cubic yard and a final pay total is shown to the nearest 0.1 cubic yard.
Cantilever and butterfly tubular support trusses shall have standard pay items. Span tubular trusses shall require special pay items. Information in the description shall include span length, truss number and span design type. Structure pay items shall include costs for all labor and materials associated with the structure, from the bottom of the base plate up, on up, as a lump sum item. Each span structure shall have a separate pay item. Structure data shall be provided on Form D-34.
All box trusses shall require a special pay item for each truss. All pay item descriptions shall include span length and truss number. Truss pay items shall include costs for all labor and materials associated with the truss, from the bottom of the base plate up, as a lump sum item. Each box truss, regardless of type, shall have a separate pay item.
See Standard Plan 903.00 for payment of delineators. Delineators shall be paid for per each on Form D-29, and include installation, bolts, post and sign.
Perforated Square Steel Tube Post Breakaway assemblies shall be totaled on Form D-29. Breakaway assemblies are incidental for pipe and structural steel posts.
Backing bar lengths and weights shall be shown on Form D-29, and are totaled with the pay item for structural steel posts. No weight deductions shall be made for punched or drilled holes. If no structural steel posts are used on a project, backing bar weights shall be added to pipe post weights.
Signal Sign Mounting Hardware shall be paid for per each on Form D-37A separate from signal signs, which will be paid for by square feet. Signal Sign Hardware will include all mounting hardware necessary to install one sign on the mast arm.
Special pay items shall not be included for items considered to be small amounts of work such as: strapping signs to lighting or signal posts or truss columns; covering inappropriate legends; "EXIT ONLY" panels on new signs; any symbol, arrow, shield or legend on new guide signs; hinge plates; aluminum wide flange posts for connecting service signs and exit number panels to structural guide signs; etc. No additional payment shall be made for hardware. Other than the above, it shall be left to the designer to decide which items require direct pay.
Option. Special pay items for signing may be required. Some examples of special work include: modifying legends, relocating existing signs to new posts, temporary ground mounting guide signs, bridge mounted support brackets, truss painting, pedestal repair, etc. It is left to the designer to decide which items require special pay items.
Support. Most jobs include the removal of existing signs and/or trusses. All removals are listed with other roadway Removal of Improvements. It is preferred to list the type of truss to be removed, number of pedestals, posts, footings and a rough estimate of sign area. Consult the District Traffic Engineer or District Constructions and Materials Engineer about which removals to salvage and where the contractor should deliver the salvaged materials. Items to be salvaged and delivery of these items are mentioned in the job special provisions and this work is paid for under Removal of Improvements.
903.17.1 Delineators
Support. Refer to Standard Plan 903.00 for delineator placement and use. Refer to EPG 620.5 for more information on delineators.
Delineators are placed on all divided highways, expressways, non-interstate freeways and interchanges. It is not necessary to spot each delineator on the sign location plan, unless the geometrics are unusual, and placement is not readily apparent when referencing the standard plans. Totals are estimated and shown on Form D-29.
903.17.5 Object Markers for Ends of Roadways (MUTCD Section 2C.66)

Support. The Type 4 object marker is used to warn and alert road users of the end of a roadway in other than construction or maintenance areas.
Standard. If object markers are used to mark the end of a roadway, four Type 4 object marker shall be used. See Standard Plan 903.00 for installation details.
Option. The Type 4 object marker may be used in instances where there are no alternate vehicular paths.
Standard. The minimum mounting height, measured vertically from the bottom of a Type 4 object marker to the elevation of the near edge of the traveled way, shall be 4 feet.
Guidance. Appropriate advance warning signs should be used.
1044.2.1 Mile and Object Marker, and Delineator Posts
The required shape, length, weight (mass) and hole punching diagram for mile marker and delineator posts may be found on Standard Plan 903.00. The inspector should be aware that minimum and maximum weights (masses) and shape dimensions found on the Standard Plan are absolute and no additional tolerance is allowed. The specified weight (mass) of these posts is before galvanizing and before fabrication. Therefore, if the posts are weighed after fabrication and galvanizing and the weight (mass) per linear ft. (meter) is found to be at the specified limit or slightly out, the weight (mass) of the steel in the post will have to be calculated by adding the theoretical weight (mass) of the steel punched out to form the holes and deducting the theoretical weight of the galvanized coating. An example calculation is available.
Field determination of weight (mass) of coating is to be made. The magnetic gauge is to be operated and calibrated in accordance with ASTM E376. A single-spot test is to be comprised of five readings of the magnetic gauge taken in a small area and averaged to obtain a single test result. Three such areas should be tested, one area near each end and one near the center. This would yield three single-spot test results for that specimen. Average the three test results to obtain the average coating weight (mass) for that specimen. Average all test results from all specimens to obtain the average coating weight (mass) to be reported. The minimum result would be the lowest average coating weight (mass) found on any one specimen. Material may be accepted or rejected for galvanized coating on the basis of magnetic gauge results. If a test result fails to comply with the specifications, that lot should be re-sampled at double the original rate. If any of the re-samples fail to comply with the specifications, that lot is to be rejected. The contractor or supplier is to be given the option of sampling for Laboratory testing if the magnetic gauge test results are within minus 15 percent of the specified coating weight (mass).
1044.5.1.2 Physical Tests
Dimensions, shape, mass, length, and hole punching shall be measured for conformance to Standard Plan 903.00. To determine the mass per linear foot (linear meter) of a galvanized steel post after fabrication, refer to 1044.3 Calculations. Test results shall be recorded through AWP.
REVISION REQUEST 4033
751.5.9.2.5 Spacing Limits
Reinforcement spacing shall be in accordance with LRFD 5.10.3, unless modified by the following criteria or elsewhere shown in the EPG.
Minimum Spacing - Moment Reinforcement | |
---|---|
Preferred Min. - Footings | 6" centers |
Preferred Min. - Slabs, Culvert Walls and Retaining Walls | 6" centers |
Absolute Min. - Slabs, Culvert Walls and Retaining Walls | 5” centers |
Preferred Min. - All Other | 4” centers |
Absolute Min. | 2 1/2” clear |
Maximum Spacing - Moment Reinforcement | |
Absolute Max. - Slabs | 1.5(slab thickness) |
Absolute Max. - All Other | 18" |
Minimum Spacing - Shear Reinforcement | |
Absolute Min. - Substructure Beams (single stirrups) | 5" centers |
Absolute Min. - Substructure Beams (double Stirrups) | 6" centers |
Absolute Min. - Prestressed Slab Beams, Box Beams and I Girders | 5" centers |
Maximum Spacing - Shear Reinforcement | |
Absolute Max. - Substructure Beams | 12" centers |
Absolute Max. - Prestressed Slab Beams, Box Beams and I Girders | Refer to EPG 751.22 P/S Concrete I Girders |
Minimum Spacing - Longitudinal Compression Reinforcement (Include 1/2-inch buffer for mechanical bar splices) | |
Absolute Min. | 4 1/2" centers (5" centers) |
Absolute Min. - Cols. (thru #10) | 2" clear (2 1/2" clear) |
Absolute Min. - Cols. (#11, #14) | 2 1/2" clear (3" clear) |
Absolute Min. - Cols (#18) | 3 1/2” clear (4" clear) |
For Drilled Shafts and Rock Sockets, see EPG 751.37.6.1 Reinforcement Design. | |
Minimum Pitch - Spiral Reinforcement for Compression Members (Static) | |
For Columns, Drilled Shafts, Rock Sockets | See EPG 751.31.3.2 Column |
Minimum Spacing- Ties (Transverse) Reinforcement for Compression Members (Static) | |
For Columns | See EPG 751.31.3.2 Column |
For Drilled Shafts and Rock Sockets, see EPG 751.37.6.1 Reinforcement Design. | 6” centers for #4 bars |
Maximum Spacing - Longitudinal Compression Reinforcement | |
Absolute Max. - the minimum number of longitudinal reinforcing bars shall be six for circular members and four for bars in a rectangular arrangement. For other requirements, see LRFD | |
Maximum Pitch - Spiral Reinforcement for Compression Members (Static) | |
Absolute Max. - Spirals | 6” pitch |
Maximum Spacing - Ties (Transverse) Reinforcement for Compression Members (Static) | |
Absolute Max. - Ties | 12" centers |
Minimum & Maximum Pitch- Spiral Reinforcement for Compression Members (Seismic) | |
See EPG 751.9.1.2 LRFD Seismic Details |
751.5.9.2.6 Cover Limits
Situation | Minimum Cover | |
---|---|---|
Concrete cast against and permanently exposed to earth: | ||
- primary reinforcement | 3" | |
- stirrups, ties, spirals | 2 1/2" | |
Conc. exposed to earth or weather: | ||
- primary reinforcement | 2" | |
- stirrups, ties, spirals | 1 1/2" | |
Conc. slabs which have no positive corrosion protection: | ||
- top reinforcement | 3" * | |
- bottom reinforcement | 1" | |
Conc. not exposed to weather or in contact with ground: | ||
- primary reinforcement (thru #11) | 1 1/2" | |
- stirrups, ties, spirals | 1" | |
Conc. piles cast against or permanently exposed to earth | 2" | |
* Absolute minimum cover shall be 2½ inches by LRFD 5.12.3. The minimum cover for stirrup and tie steel shall be 1½ inches unless otherwise specified. For minimum cover for drilled shafts and rock sockets, see EPG 751.37.6.1 Reinforcement Design. |
751.9.1.2 LRFD Seismic Details
751.9.1.2.1 Seismic Details for Column Supported on Footing
Seismic Design Category, SDC B | |||||||||||
---|---|---|---|---|---|---|---|---|---|---|---|
Shear Reinf. |
Diameter (inch) |
Min. cover (inch) |
Core D' (inch) |
spiral/hoop size1 | Area of spiral/hoop bar Asp (sq. inch) |
Pitch or space s (inch) |
f'c (ksi) |
Ro = 4Asp/(D'*s) SGS Eq 8.6.2‐7 |
Ro min SGS 8.6.5 |
||
Spiral | 36 | 1.5 | 32.375 | 5 | 0.307 | 4 | 3 | 0.0095 | ≥ | 0.003 | OK |
Spiral | 42 | 1.5 | 38.375 | 5 | 0.307 | 4 | 3 | 0.0080 | ≥ | 0.003 | OK |
Spiral | 48 | 1.5 | 44.375 | 5 | 0.307 | 4 | 3 | 0.0069 | ≥ | 0.003 | OK |
Spiral | 54 | 1.5 | 50.375 | 5 | 0.307 | 4 | 3 | 0.0061 | ≥ | 0.003 | OK |
Hoop | 60 | 1.5 | 56.375 | 5 | 0.307 | 4 | 3 | 0.0054 | ≥ | 0.003 | OK |
Hoop | 66 | 1.5 | 62.375 | 5 | 0.307 | 4 | 3 | 0.0049 | ≥ | 0.003 | OK |
Hoop | 72 | 1.5 | 68.375 | 5 | 0.307 | 4 | 3 | 0.0045 | ≥ | 0.003 | OK |
Seismic Design Category, SDC C and D | |||||||||||
---|---|---|---|---|---|---|---|---|---|---|---|
Shear Reinf. |
Diameter (inch) |
Min. cover (inch) |
Core D' (inch) |
spiral/hoop size1 | Area of spiral/hoop bar Asp (sq. inch) |
Pitch or space s (inch) |
f'c (ksi) |
Ro = 4Asp/(D'*s) SGS Eq 8.6.2‐7 |
Ro min SGS 8.6.5 |
||
Spiral | 36 | 1.5 | 32.375 | 5 | 0.307 | 4 | 3 | 0.0095 | ≥ | 0.005 | OK |
Spiral | 42 | 1.5 | 38.375 | 5 | 0.307 | 4 | 3 | 0.0080 | ≥ | 0.005 | OK |
Spiral | 48 | 1.5 | 44.375 | 5 | 0.307 | 4 | 3 | 0.0069 | ≥ | 0.005 | OK |
Spiral | 54 | 1.5 | 50.375 | 5 | 0.307 | 4 | 3 | 0.0061 | ≥ | 0.005 | OK |
Hoop | 60 | 1.5 | 56.375 | 5 | 0.307 | 4 | 3 | 0.0054 | ≥ | 0.005 | OK |
Hoop | 66 | 1.5 | 62.25 | 6 | 0.442 | 4 | 3 | 0.0071 | ≥ | 0.005 | OK |
Hoop | 72 | 1.5 | 68.25 | 6 | 0.442 | 4 | 3 | 0.0065 | ≥ | 0.005 | OK |
Note: | 1For simplification use minimum #5 spiral/hoop bar. | SGS 8.8.9 |
Ro shall be ≥ 0.003 in SDC B and 0.005 in SDC C and D. No need to meet LRFD 5.6.4.6‐1 & 5.11.4.1.4‐1 minimum Ro requirements. | SGS 8.6.5 | |
Use 4" spiral pitch/hoop spacing for column to meet long. bar splice area requirements. | LRFD 5.11.4.1.6 | |
Use spiral or hoop but combination of spiral reinforcement with hoops shall not be used except in the footing or bent cap. | SGS 8.8.7 | |
Closed tie (Hoop) shall use 135‐degree hook with an extension of 6 bar diameters but not less than 3". | SGS 8.8.9 | |
Welding of reinforcing steel (spiral, hoop and longitudinal) is not permitted due to the prohibitive cost of weld inspection. | ||
Spiral does not need to meet end tail requirements of SGS 8.8.7. | ||
(1) Anchorage of spiral reinforcement shall be provided by 1 1/2 extra turns of spiral reinforcement at end of the spiral unit. | ||
(2) Lac = max(Lac from SGS 8.8.4, 1.25 Ld) or Ldh, but shall be extended to the clear cover specified herein. | ||
(3) 11 inches for #8 thru #11 bars and 14 inches for #14 bars. | ||
(4) Plastic hinge area for SDC B: Lpr ≥ max(1.0 * col dia, 1/6 clear col ht., 18") | SGS C8.8.9 & LRFD C5.11.4.1.4 | |
(4) Plastic hinge area for SDC C and D: Lpr ≥ max(1.5 * col dia, Lp, 1/6 clear column ht.) | SGS 4.11.6 and 4.11.7 | |
(4) Long reinf. and spiral bar shall not be spliced in plastic hinge area. If splice is unavoidable, a mechanical bar splice shall be used. | SGS 8.8.3 LRFD 5.11.4.1.6 | |
(5) Minimum lap: Use greater of EPG 751.5.9.2.8.2 Class B lap splice or 60 bar diameters. Lap splices and mechanical bar splices are to be alternately staggered at least 24”at two different locations. | LRFD 5.10.8.4.3b | |
For dowel bar in beam cap, See EPG 751 .22.2.7 Dowel Bars | ||
For additional requirements of column joints in SDC C and D, See EPG 751 .9.1.2.4 T-Joint (Column Joint) Connections for Seismic Design Category C and D. | ||
Use Figure 751.9.1.2.1.1 and Figure 751.9.1.2.1.2 for seismic detail option. For complete seismic design option spiral/hoop bar size shall be increased up to #6 and pitch/spacing shall be reduced as needed by design. Absolute minimum clearance is 1.5 inches. |
-
Figure 751.9.1.2.1.1 Seismic Details for Column Supported on Footing
-
Figure 751.9.1.2.1.2 Seismic Bar Details
751.9.1.2.2 Seismic Details for Non-oversized Drilled Shaft
Seismic Design Category, SDC B | ||||||||||||
---|---|---|---|---|---|---|---|---|---|---|---|---|
Shear Reinf. |
Diameter (inch) |
Min. cover (inch) |
Core D' (inch) |
spiral/hoop size1 | 1 for single bar 2 for bundle hoop bars |
Area of spiral/hoop bar Asp (sq. inch) |
Pitch or space s (inch) |
f'c (ksi) |
Ro = 4Asp/(D'*s) SGS Eq 8.6.2‐7 |
Ro min SGS 8.6.5 |
||
Spiral | 36 | 6 | 23.375 | 5 | 1 | 0.307 | 6 | 4 | 0.0087 | ≥ | 0.003 | OK |
Spiral | 42 | 6 | 29.375 | 5 | 1 | 0.307 | 6 | 4 | 0.0070 | ≥ | 0.003 | OK |
Spiral | 48 | 6 | 35.375 | 5 | 1 | 0.307 | 6 | 4 | 0.0058 | ≥ | 0.003 | OK |
Spiral | 54 | 6 | 41.375 | 5 | 1 | 0.307 | 6 | 4 | 0.0049 | ≥ | 0.003 | OK |
Spiral | 60 | 6 | 47.375 | 5 | 1 | 0.307 | 6 | 4 | 0.0043 | ≥ | 0.003 | OK |
Hoop | 66 | 6 | 53.375 | 5 | 1 | 0.307 | 6 | 4 | 0.0038 | ≥ | 0.003 | OK |
Hoop | 72 | 6 | 59.375 | 5 | 1 | 0.307 | 6 | 4 | 0.0034 | ≥ | 0.003 | OK |
Hoop | 78 | 6 | 65.25 | 6 | 1 | 0.442 | 6 | 4 | 0.0045 | ≥ | 0.003 | OK |
Seismic Design Category, SDC C and D | ||||||||||||
---|---|---|---|---|---|---|---|---|---|---|---|---|
Shear Reinf. |
Diameter (inch) |
Min. cover (inch) |
Core D' (inch) |
spiral/hoop size1 | 1 for single bar 2 for bundle hoop bars |
Area of spiral/hoop bar Asp (sq. inch) |
Pitch or space s (inch) |
f'c (ksi) |
Ro = 4Asp/(D'*s) SGS Eq 8.6.2‐7 |
Ro min SGS 8.6.5 |
||
Spiral | 36 | 6 | 23.375 | 5 | 1 | 0.307 | 6 | 4 | 0.0087 | ≥ | 0.005 | OK |
Spiral | 42 | 6 | 29.375 | 5 | 1 | 0.307 | 6 | 4 | 0.0070 | ≥ | 0.005 | OK |
Spiral | 48 | 6 | 35.375 | 5 | 1 | 0.307 | 6 | 4 | 0.0058 | ≥ | 0.005 | OK |
Spiral | 54 | 6 | 41.25 | 6 | 1 | 0.442 | 6 | 4 | 0.0071 | ≥ | 0.005 | OK |
Spiral | 60 | 6 | 47.25 | 6 | 1 | 0.442 | 6 | 4 | 0.0062 | ≥ | 0.005 | OK |
Hoop | 66 | 6 | 53.25 | 6 | 1 | 0.442 | 6 | 4 | 0.0055 | ≥ | 0.005 | OK |
Hoop | 72 | 6 | 59.375 | 5 | 2 | 0.614 | 8 | 4 | 0.0052 | ≥ | 0.005 | OK |
Hoop | 78 | 6 | 65.25 | 6 | 2 | 0.884 | 8 | 4 | 0.0068 | ≥ | 0.005 | OK |
Note: | 1For simplification use minimum #5 spiral/hoop bar. | SGS 8.8.9 |
Ro shall be ≥ 0.003 in SDC B and 0.005 in SDC C and D. No need to meet LRFD 5.6.4.6‐1 & 5.11.4.1.4‐1 minimum Ro requirements. | SGS 8.6.5 | |
Closed tie (Hoop) shall use 135‐degree hook with an extension of 6 bar diameters but not less than 3". | SGS 8.8.9 | |
Spiral does not need to meet end tail requirements of SGS 8.8.7. | ||
(6) Anchorage of spiral reinforcement shall be provided by 1 1/2 extra turns of spiral reinforcement at end of the spiral unit. | ||
(7) Plastic hinge area for SDC B: Lpr ≥ drilled shaft diameter. | SGS C8.8.9 and LRFD C5.11.4.1.4 | |
(7) Plastic hinge area for SDC C and D: Lpr ≥ max(1.5 * Column dia., Lp, drilled shaft diameter). | SGS 4.11.6 and 4.11.7 | |
(7,8) Long reinforcement and spiral bar shall not be spliced in plastic hinge area. If splice is unavoidable, a mechanical bar splice shall be used. | SGS 8.8.3 and LRFD 5.11.4.1.6 | |
(8) Plastic hinge area : Lpr ≥ drilled shaft diameter. | ||
(9) Minimum lap: Use greater of EPG 751.5.9.2.8.2 Class B lap splice or 60 bar diameters. Lap splices and mechanical bar splices are to be alternately staggered at least 24”at two different locations. | LRFD 5.10.8.4.3b | |
(10) Use spiral or hoop but combination of spiral reinforcement with hoops shall not be used except in the bent cap. From above table if hoop required for drilled shaft than hoop shall be used in the column and if spiral required for drilled shaft than spiral shall be used in column. Use spiral or hoop bar size and pitch or spacing per EPG 751.9.1.2.1 | SGS 8.8.7 | |
Welding of reinforcing steel (spiral, hoop and longitudinal) is not permitted due to the prohibitive cost of weld inspection. | ||
For detail simplification consider drilled shaft 6” larger than column. Avoid sizing shafts 12” larger than column. | ||
For column and beam detail requirements, see EPG 751.9.1.2.1. | ||
For oversized shaft (generally 18" minimum larger than column), see EPG 751.9.1.2.3 Seismic Details for Oversized Drilled Shaft. | ||
For additional requirements of column joints in SDC C and D, See EPG 751.9.1.2.4 T-Joint (Column Joint) Connections for Seismic Design Category C and D. | ||
Use Figure 751.9.1.2.2 for seismic detail option. For complete seismic design option spiral/hoop bar size shall be increased up to #6 and pitch/spacing shall be 6” by design. Absolute minimum clearance is 5 inches. If #6 at 6” spiral or hoop do not meet design requirements, then use 2-#6 hoop bars @ 8” spacing. | ||
For seismic bar details (spiral and hoop), see Figure 751.9.1.2.1.2 |
-
Figure 751.9.1.2.2 Seismic Details for Non-oversized Drilled Shaft
751.9.1.2.3 Seismic Details for Oversized Drilled Shaft
Seismic Design Category, SDC B | ||||||||||||
---|---|---|---|---|---|---|---|---|---|---|---|---|
Shear Reinf. |
Diameter (inch) |
Min. cover (inch) |
Core D' (inch) |
spiral/hoop size1 | 1 for single bar 2 for bundle hoop bars |
Area of spiral/hoop bar Asp (sq. inch) |
Pitch or space s (inch) |
f'c (ksi) |
Ro = 4Asp/(D'*s) SGS Eq 8.6.2‐7 |
Ro min SGS 8.6.5 |
||
Spiral | 36 | 6 | 23.375 | 5 | 1 | 0.307 | 6 | 4 | 0.0087 | ≥ | 0.003 | OK |
Spiral | 42 | 6 | 29.375 | 5 | 1 | 0.307 | 6 | 4 | 0.0070 | ≥ | 0.003 | OK |
Spiral | 48 | 6 | 35.375 | 5 | 1 | 0.307 | 6 | 4 | 0.0058 | ≥ | 0.003 | OK |
Spiral | 54 | 6 | 41.375 | 5 | 1 | 0.307 | 6 | 4 | 0.0049 | ≥ | 0.003 | OK |
Spiral | 60 | 6 | 47.375 | 5 | 1 | 0.307 | 6 | 4 | 0.0043 | ≥ | 0.003 | OK |
Spiral/Hoop | 66 | 6 | 53.375 | 5 | 1 | 0.307 | 6 | 4 | 0.0038 | ≥ | 0.003 | OK |
Spiral/Hoop | 72 | 6 | 59.375 | 5 | 1 | 0.307 | 6 | 4 | 0.0034 | ≥ | 0.003 | OK |
Spiral/Hoop | 78 | 6 | 65.25 | 6 | 1 | 0.442 | 6 | 4 | 0.0045 | ≥ | 0.003 | OK |
Seismic Design Category, SDC C and D | ||||||||||||
---|---|---|---|---|---|---|---|---|---|---|---|---|
Shear Reinf. |
Diameter (inch) |
Min. cover (inch) |
Core D' (inch) |
spiral/hoop size1 | 1 for single bar 2 for bundle hoop bars |
Area of spiral/hoop bar Asp (sq. inch) |
Pitch or space s (inch) |
f'c (ksi) |
Ro = 4Asp/(D'*s) SGS Eq 8.6.2‐7 |
Ro min SGS 8.6.5 |
||
Spiral | 36 | 6 | 23.375 | 5 | 1 | 0.307 | 6 | 4 | 0.0087 | ≥ | 0.005 | OK |
Spiral | 42 | 6 | 29.375 | 5 | 1 | 0.307 | 6 | 4 | 0.0070 | ≥ | 0.005 | OK |
Spiral | 48 | 6 | 35.375 | 5 | 1 | 0.307 | 6 | 4 | 0.0058 | ≥ | 0.005 | OK |
Spiral | 54 | 6 | 41.25 | 6 | 1 | 0.442 | 6 | 4 | 0.0071 | ≥ | 0.005 | OK |
Spiral | 60 | 6 | 47.25 | 6 | 1 | 0.442 | 6 | 4 | 0.0062 | ≥ | 0.005 | OK |
Spiral/Hoop | 66 | 6 | 53.25 | 6 | 1 | 0.442 | 6 | 4 | 0.0055 | ≥ | 0.005 | OK |
Hoop | 72 | 6 | 59.375 | 5 | 2 | 0.614 | 8 | 4 | 0.0052 | ≥ | 0.005 | OK |
Hoop | 78 | 6 | 65.25 | 6 | 2 | 0.884 | 8 | 4 | 0.0068 | ≥ | 0.005 | OK |
Note: | 1For simplification use minimum #5 spiral/hoop bar. | SGS 8.8.9 |
Ro shall be ≥ 0.003 in SDC B and 0.005 in SDC C and D. No need to meet LRFD 5.6.4.6‐1 & 5.11.4.1.4‐1 minimum Ro requirements. | SGS 8.6.5 | |
Closed tie (Hoop) shall use 135‐degree hook with an extension of 6 bar diameters but not less than 3". | SGS 8.8.9 | |
Spiral does not need to meet end tail requirements of SGS 8.8.7. | ||
(11) Anchorage of spiral reinforcement shall be provided by 1 1/2 extra turns of spiral reinforcement at end of the spiral unit. | ||
(12) Plastic hinge area for SDC B: Lpr ≥ drilled shaft diameter. | SGS C8.8.9 and LRFD C5.11.4.1.4 | |
(12) Plastic hinge area for SDC C and D: Lpr ≥ max(1.5 * Column dia., Lp, drilled shaft diameter). | SGS 4.11.6 and 4.11.7 | |
(12) Long reinforcement and spiral bar shall not be spliced in plastic hinge area. If splice is unavoidable, a mechanical bar splice shall be used. | SGS 8.8.3 and LRFD 5.11.4.1.6 | |
(13) Plastic hinge area : Lpr ≥ drilled shaft diameter. | ||
(14) Minimum lap: Use greater of EPG 751.5.9.2.8.2 Class B lap splice or 60 bar diameters. Lap splices and mechanical bar splices are to be alternately staggered at least 24”at two different locations. | LRFD 5.10.8.4.3b | |
(15) Since column reinforcement embedded into drilled shaft, clear spacing between column reinforcement shall be 5” min. | ||
(16) Spiral pitch or hoop bar spacing shall be same as drilled shaft requirements. | ||
Use spiral or hoop but combination of spiral reinforcement with hoops shall not be used in a reinforcement cage except in bent cap. Spirals in the column cage and hoops in the drilled shaft cage can be used for oversized drilled shaft | SGS 8.8.7 | |
Welding of reinforcing steel (spiral, hoop and longitudinal) is not permitted due to the prohibitive cost of weld inspection. | ||
Hoops are preferred for drilled shafts with diameters at least 2’-6” larger than column. Spirals shall be used in drilled shafts that are oversized by 18” or 24” due to potential interference between the hooks and the column reinforcing cage. | ||
Column confinement: | ||
For column use spiral/pitch or hoop/spacing and bar size per column shear reinforcement requirements. | ||
Drilled shaft confinement: | ||
Exterior cage: From above table use spiral/pitch or hoop/spacing for drilled shaft. | ||
Interior cage: Column confinement reinforcement bar size from column shear reinforcement table shall be spaced or pitched same as drilled shaft and provided over entire embedded length of column steel in drilled shaft. |
||
For column and beam detail requirements, see column shear reinforcement. | ||
For additional requirements of column joints in SDC C and D, See EPG 751.9.1.2.4 T-Joint (Column Joint) Connections for Seismic Design Category C and D. | ||
Use Figure 751.9.1.2.3 for seismic detail option. For complete seismic design option spiral/hoop bar size shall be increased up to #6 and pitch/spacing shall be 6” by design. Absolute minimum clearance is 5 inches. If #6 at 6” spiral or hoop do not meet design requirements, then use 2-#6 hoop bars @ 8” spacing. | ||
For seismic bar details (spiral and hoop), see Figure 751.9.1.2.1.2 |
-
Figure 751.9.1.2.3 Seismic Details for Oversized Drilled Shaft
751.9.1.2.4 T-Joint (Column Joint) Connections for Seismic Design category C and D
Minimum joint reinforcement shall be provided as shown below. Reinforcement marked as “additional” shall not be used to satisfy other load requirements.
751.9.1.2.4.1 Bent Cap Joint Shear Reinforcement
1. | Additional Vertical Stirrups Outside the Joint Region | SGS 8.13.5.1.1 |
Total area of column longitudinal reinforcement anchored in the joint = Total area of column longitudinal reinforcement anchored in the joint | ||
Minimum total area of additional vertical stirrups on each side of joint | ||
Total area of vertical stirrups shall be provided transversely within a distance equal to the column diameter extending from each face of the column as shown in Figure 751.9.1.2.4.1a. For additional vertical stirrups size and spacing limitations, see EPG 751.31.3.1. | ||
2. | Additional Vertical Stirrups Inside the Joint Region | SGS 8.13.5.1.2 |
Where, | ||
Total area of column longitudinal reinforcement anchored in the joint | ||
Minimum total area of vertical stirrups inside the joint region | ||
Total area of vertical stirrups spaced evenly over the column inside the joint region as shown in Figure 751.9.1.2.4.1a. For additional vertical stirrups size and spacing limitations, see EPG 751.31.3.1. | ||
3. | Additional Longitudinal Cap Beam Reinforcement | SGS 8.13.5.1.3 |
Where, | ||
Total area of column longitudinal reinforcement anchored in the joint | ||
Minimum total area of additional longitudinal reinforcement in top and bottom faces of the cap beam | ||
The additional longitudinal reinforcement shall be extended at least one column diameter plus development length from face of the column as shown in Figure 751.9.1.2.4.1a | ||
4. | Horizontal J-Bars | SGS 8.13.5.1.4 |
#4 Horizontal J-bars shall be hooked around the longitudinal reinforcement on each face of the cap beam at every other stirrup inside the joint as shown in Figure 751.9.1.2.4.1b. | ||
When complete seismic analysis is required per bridge seismic design flowchart, joint shear reinforcement shall be provided in accordance with AASHTO Guide Specifications for LRFD Seismic Bridge Design (SGS). Modify above information and provide additional reinforcement as needed by design. | SGS 8.12 and 8.13 |
-
Figure 751.9.1.2.4.1a Elevation Showing Column and Beam Cap Reinforcement
(SDC C and D only)
-
Figure 751.9.1.2.4.1b Section Showing Column and Beam Cap Reinforcement
(SDC C and D only)
751.9.1.2.4.2 Footing (Spread Footing and Pile Cap Footing) Joint Shear Reinforcement
For seismic detail option, use following information for spread footing and pile cap footing | SGS 6.4.7 | ||
Vertical shear reinforcement #5 at about 12” each way shall be placed around the column perimeter within a horizontal dimension from face of the column equal to minimum as shown in Figure 751.9.1.2.4.2a and Figure 751.9.1.2.4.2b. #6 maximum bar size shall be used for vertical shear reinforcement. | |||
Effective depth from top of footing to lower reinforcement mat. | |||
Additional longitudinal horizontal reinforcement at top of footing, : | |||
= | |||
Where, | |||
= Total area of column longitudinal reinforcement anchored in the joint | |||
= Expected yield stress of column longitudinal reinforcement | |||
= 68 ksi for ASTM A706 and ASTM A615 | SGS Table 8.4.2-1 | ||
= Minimum yield stress of column longitudinal reinforcement | |||
= 60 ksi for ASTM A706 and ASTM A615 | SGS Table 8.4.2-1 | ||
The additional longitudinal reinforcement shall be extended at least up to a distance plus development length from face of the column and must be placed so that the reinforcement goes through the column reinforcement as shown in Figure 751.9.1.2.4.2b shall be provided in both directions in the footing. | |||
When complete seismic analysis is required per bridge seismic design flowchart, joint shear reinforcement shall be provided in accordance with AASHTO Guide Specifications for LRFD Seismic Bridge Design (SGS). Modify above information and provide additional reinforcement as needed by design. | SGS 6.4.5, 6.4.6 and 6.4.7 |
-
Figure 751.9.1.2.4.2a Spread Footing Joint Shear Reinforcement
-
Figure 751.9.1.2.4.2b Pile Footing Joint Shear Reinforcement
751.9.3.1.7 T- Joint Connections for LFD
For LFD T-Joint connection requirement, see EPG 751.40.8.11.5 T- Joint Connections.
!!! MOVE TO NEW ARTICLE NUMBER DARREN CHECK THIS SECTION AND MAKE SURE I FOUND AND FIXED ALL THE FIGURE NUMBERS !!!
751.40.8.11.5 T- Joint Connections
Principal Tension and Compression Stresses in Beam-Column Joints
The connections where columns and beams join, or where columns and footings join, should be based on the capacity design for shear and diagonal tension. For most locations, this is a “T”-shaped joint. For the analysis of “knee joints”, see Priestley and Seible, 1996.

In the capacity design of connection joints, the column moment, M0, will be the moment that is known and which will correspond to flexural overstrength of the column plastic hinges, i.e. M0 = 1.3Mp of the column. If the columns are designed based on plastic hinging, the beam and footings shall be designed with capacities greater than or equal to 1.3Mp.
At each joint, the principal tension and compression stresses are defined and checked as follows:
- (1)
- (2)
- (3)
- (4)
- (5)
- (6)
- (7)
- (8)
- in which:
- Vjh = Average horizontal shear force within a joint.
- Vjv = Average vertical shear force within a joint.
- vjh = Average horizontal shear stress within a joint.
- vjv = Average vertical shear stress within a joint.
- hb = Beam depth.
- hc = Column diameter or rectangular column cross-section height.
- bje = The effective width of a joint, defined in Fig. 751.40.8.11.5.2.
- D = Round column diameter.
- fv = Average vertical axial stress due to column axial force Pc, including the seismic component.
- Pc = Column axial force.
- fh = Average horizontal axial stress at the center of the joint.
- pc = Nominal principal compression stress in a joint. (positive)
- pt = Nominal principal tensile stress in a joint. (negative)
- bb = Beam width
- bc = Column cross-section width
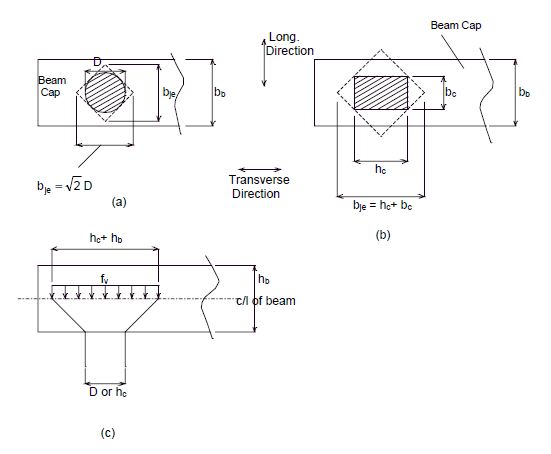
In Fig. 751.40.8.11.5.2(c), the effective width is taken at the center of the column section, allowing a 45° spread from boundaries of the column section into the beam cap. In the transverse direction, the effective width will be the smaller of the value given by eq. (3) and the beam cap width bb. Experimental evidence indicates that diagonal cracking is initiated in the joint region when psi. The principle compression stress pc shall be limited to .
Design of Reinforcement for Beam-Column Joints
When the principal tension stress, pt, exceeds psi, joint cracking occurs and the following reinforcement shall be provided:
a) Vertical beam stirrup reinforcement shall be placed throughout the distance of hb/2 from the column face on each side of the column. The required amount of vertical beam stirrup reinforcement, Ajv, is:
- (9)
- Where:
- Asc = The total area of longitudinal steel
- f°yc = overstrength stress in the column reinforcement use
- f°yc = 1.1f
- fyv = yield stress of vertical stirrup reinforcement.
b) Vertical beam stirrup reinforcement within the joint, Avi, is
- (10)
c) The additional beam bottom longitudinal reinforcement required is
- (11)
- where fyb = the yield stress of the beam bottom longitudinal reinforcement. This additional reinforcement must be carried a sufficient distance to develop its yield strength a distance hb/2 from the column face.
d) The horizontal hoop reinforcement within a joint requires
- (12)
which for F = 0 simplifies to
- (13)
- Where
- F = The beam cap prestress force.
- fyh = The yield stress of the horizontal hoops.
- La = The Anchorage length in the joint.
The minimum amount of horizontal hoop reinforcement shall be
- (14)
The spacing of the horizontal hoop can be based on:
(15)
- Where
- As = The cross-sectional area of the hoop bar.
- D’ = The hoop diameter.
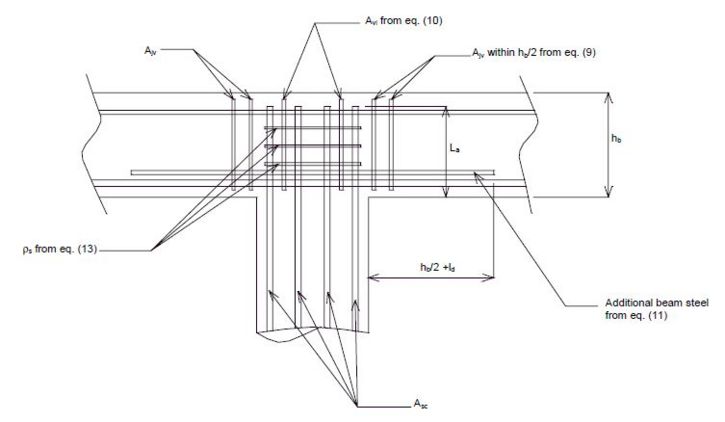
When the principal tension stress, pt, does not exceed psi, no joint cracking is expected. However, the following minimum reinforcement shall be provided:
- a) Vertical beam stirrup reinforcement within the joint based on eq. (10)
- b) Minimum horizontal hoop reinforcement based on eq. (14)
Note that the bending of any hooked reinforcement outward, away from the column core, shall not be used because it directs the anchorage force away from the joint. Inward bending of the column reinforcement is allowed. However, it is likely to cause a congestion problem. The use of straight column reinforcement embedded into the beam-column joint is recommended. The standard T-joint reinforcement details are shown in Figs. 751.40.8.11.5.4 - 751.40.8.11.5.6. If any reinforcement requirement based on eqs. (9) through (14) is greater than that shown in Figs. 751.40.8.11.5.4 - 751.40.8.11.5.6, the greater requirement shall be used.
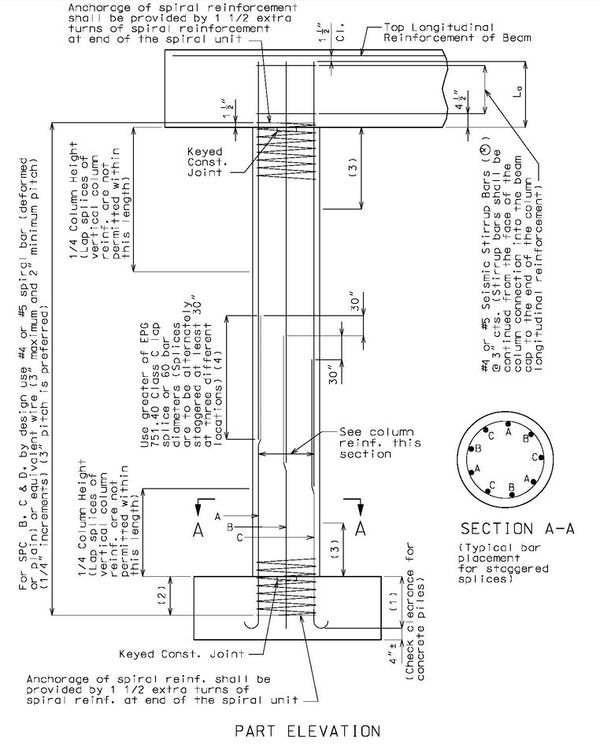
- (1) Increase by 25% the development length (other than top bars) or the standard hook in tension “Ldh” of EPG 751.40.8.4.2.
- (2) The spiral bars or wire shall be continued for a distance equal to ½ the column diameter but not less than 15” from the face of the column connection into the footing.
- (3) Use the greatest length of the following: column diameter of 1/6 of the clear height of column. Lapping of spiral reinforcement in this region is not permitted.
- (4) Splices may be eliminated when the column height is 20’-0” or less or restrictions do not practically allow for lap splices.
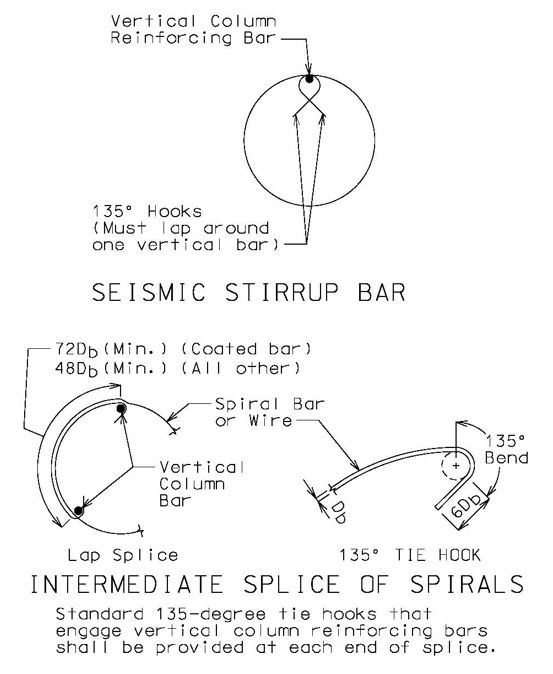
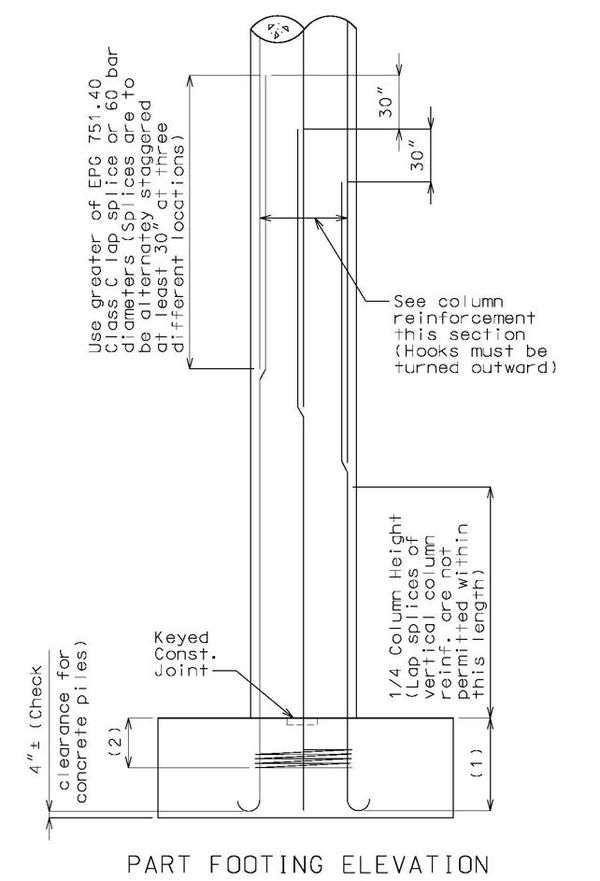
- See additional guidance in EPG 751.9.3.1.7, below, for footing reinforcement not shown.
- (1) Increase by 25% the development length (other than top bars) or the standard hook minimum in tension “Ldh” of EPG 751.40.8.4.2.
- (2) The spirals shall be continued for a distance equal to ½ the column diameter but not less than 15” from the face of the column connection into the footing.
Example 751.9.3.1.7.1: A column is subjected to an axial load (due to dead and seismic earthquake loads) of 520 kips. The column diameter is 36 in. with 20 #8 bars for longitudinal reinforcement. The beam cap dimensions are 3 ft. 9 in. wide by 3 ft. 7 in. deep with 5 #11 bars for the top reinforcement and 7 #10 bars for the bottom reinforcement as shown in Fig. 751.40.8.11.5.7. The column overstrength moment-axial load curve is shown in Fig. 751.40.8.11.5.8. Design the reinforcement details for the beam-column joint.
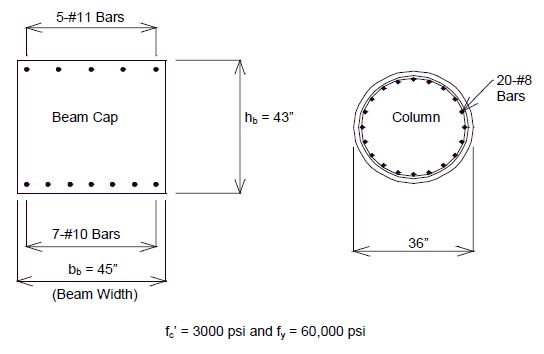
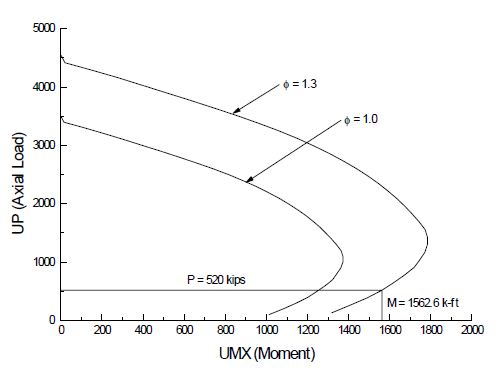
Solution:
The axial load for the column = 520 kips.
From Fig. 751.40.8.11.5.8, M0 = 1562.6 k-ft.
From eq. (1): = 436.07 kips
From eq. (3): = 45 in.
From eq. (2): = 269.18 psi.
Vertical Axial Stress:
From eq. (6):
- = 146.27 psi.
Horizontal Stress:
- fh = 0 psi.
From eq. (7):
pc = 352.07 psi ≤ 0.3(3000 psi) = 900 psi O.K.
From eq. (8):
pt = -205.80 psi ≥ 3.5 3000 = 191.7 psi Not O.K.
Since pt is greater than , special joint reinforcement based on eqs. (9) through (14) are needed.
Check if moment capacity of the beam is greater than the overstrength moment capacity of the column.
Neglect the effect of the compression steel (conservative).
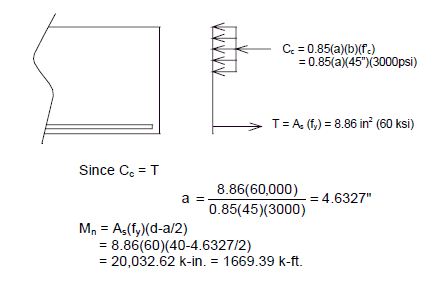
Compare moment capacity of beam versus overstrength moment capacity of the column:
- 1669.39 k-ft. > 1562.60 k-ft.
Moment capacity of beam is greater than the overstrength moment capacity of the column. O.K.
Design of reinforcement for the beam-column joint
- - Vertical reinforcement should be placed throughout a distance of hb/2 from the column face on each side of the column.
- From eq.(9):
- Asc = 15.70 in2
- From eq.(9):
- = 1.1f = 66 ksi.
- fyv = 60 ksi.
- Ajv = 0.125 (15.70) 66 / 60 = 2.16 in2
- - Reinforcement within the joint confines:
- From eq. (10):
- = 0.0625 (15.70) 66 / 60 = 1.08 in2
- From eq. (10):
- - Additional bottom of beam longitudinal reinforcement:
- From eq. (11):
- = 0.0625 (15.70) 66 / 60 = 1.08 in2
- From eq. (11):
- This reinforcement must be developed at a distance hb/2 away from the face of the column.
- - Hoop Reinforcement:
- From eq. (13):
- La = 40 in.
- fyh = 60 ksi
- ∴use ρs
- use #4 hoop reinforcement
- As = 0.1963 in2
- D’ = 36 – 2(2) – 4/8 = 31.5 in.
- ρs = 0.003238
- From eq. (15): = 7.70” spacing > 3” max. from Fig. 751.40.8.11.5.4. Therefore, Use S = 3”
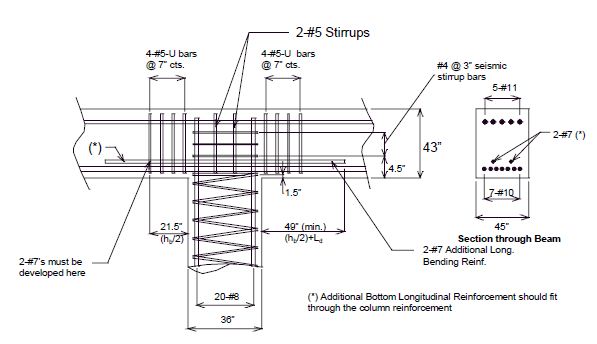
Principal Tension and Compression Stresses in Column-Footing Joints
Column – Footing joints are essentially the same as inverted beam-column T joints. Eqs. (1) through (8) are applicable to column-footing joints except the beam height, hb, shall be changed to the footing height, hf.
Design of Reinforcement for Column-Footing Joint
The design of the reinforcement for column-footing joints is similar to that for beam-column T joint. From a joint performance viewpoint, it is desirable to bend the column bars inward toward the joint by using 90° hook bars, but this will cause undue congestion. Bending column bars away from the joint will increase the diagonal tension stress within the joint region. However, it makes a stable platform for supporting the column cage and prevents congestion. When the column reinforcement is bent outward, eqs. (9) through (14) shall be applied. Since the column inelastic action may develop in directions other than parallel to one of the principal axes of the footing, the amount of vertical reinforcement in eq. (9) shall be placed in each of the four quadrant areas outside the joint. In other words, a total vertical stirrup area of:
- (16)
shall be placed around the column.
Extra top reinforcement in the footing is also required in accordance with eq. (11). This reinforcement should pass through the column reinforcement or be placed as close as possible to the sides of the column and extend a distance of not less than l = 0.5*D + Ld, where Ld is the bar development length, beyond the face on both sides of the column.
Example 751.9.3.1.7.2: A column is subjected to an axial load (due to dead and seismic loads) of 520 kips. The column diameter is 36 in. with 20 #8 bars for longitudinal reinforcement. All column reinforcement is bent outward into the footing away from the joint. The footing depth is 39 inches. The top and bottom reinforcement for the footing is shown in Fig. 751.40.8.11.5.10, below. Design the reinforcement details for the column-footing joint.
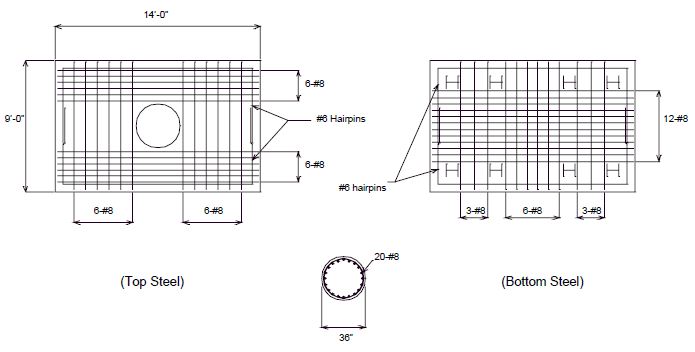
Solution:
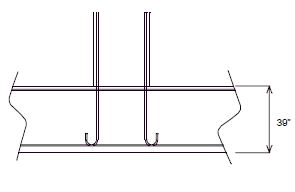
The axial load for the column = 520 kips.
From Fig. 751.40.8.11.5.8 in Example 751.9.3.1.7.1, M0 = 1562.6 k-ft.
From eq.(1): = 480.8 kips
From eq. (3):
From eq. (2): =262.39 psi.
Vertical Axial Stress:
From eq. (6):
- = 136.21psi.
Horizontal Axial Stress:
- fh = 0 psi.
Principal Stresses:
From eq. (7):
- = 339.19 psi. ≤ 0.3(3000psi.) = 900 psi. O.K.
From eq. (8):
- = -202.98 psi. > 3.5 = 191.7 psi. Not O.K.
Since pt is greater than allowed, special joint reinforcement based on eqs. (9)through (14) are needed.
Check moment capacity
Check the moment capacity of footing in the long direction to see if it is greater than the overstrength moment capacity of the column.
Neglect the effect of the compression reinforcement.
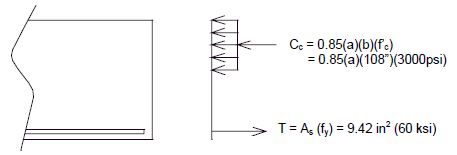
- Since Cc = T
- = 2.0523"
- Mn = As(fy)(d - a/2)
- = 9.42(60)(35-(2.0523/2))
- = 1600.17 k-ft.
Compare moment capacity of footing overstrength moment capacity of the column:
- 1600.17 k-ft > 1562.60 k-ft.
Moment capacity of the footing is greater than the overstrength moment of capacity of the column. O.K.
Check the moment capacity of the footing in the short direction to see if it is greater than the overstrength moment capacity of the column.
Neglect the effect of the compression reinforcement.
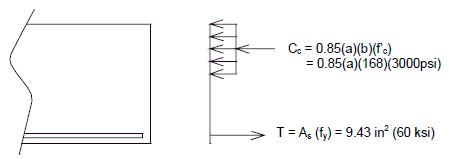
- Since Cc = T
- = 1.3207"
- Mn = As(fy)(d - a/2)
- = 9.43(60)(35-(1.3207/2))
- = 1619.11 k-ft.
Compare moment capacity of footing overstrength moment capacity of the column:
- 1619.11 k-ft > 1562.60 k-ft.
Moment capacity of the footing is greater than the overstrength moment of capacity of the column. O.K.
Design of reinforcement for the column-footing joint
- - Vertical reinforcement should be placed throughout a distance of hf/2 from the column face on each side of the column.
- From eq. (16):
- Asc = 15.71 in2
- = 1.1 fy = 66 ksi.
- fyv = 60 ksi.
- Ajv = 0.5 (15.71) (66 / 60) = 8.641 in2
- From eq. (16):
- - Reinforcement within the joint confines:
- From eqs. (9),(10) & (16):
-
- = 0.25 (15.71)(66/60) = 4.320 in2
-
- From eqs. (9),(10) & (16):
- - Additional top of footing longitudinal reinforcement:
-
- = 0.0625 (15.70)(66 / 60) = 1.08 in2
-
- This reinforcement must be developed at a distance hb/2 away from the face of the column and must be placed so that the reinforcement goes through the column reinforcement. Asb is required in both directions in the footing.
- - Hoop Reinforcement:
- From eq. (13):
- La = 35 in.
- fyh = 60 ksi
- = 0.004232
- = 0.003195
- ρs > ρs, min ∴use ρs
- Use #5 hoop reinforcement
- As = 0.3068
- D’ = 36 - 2(2) - 5/8” = 31.375”
- ρs = 0.004232
- From eq. (15): = 9.24"
- Use 9” Spacing
- Note: By adding 3 in. to footing depth in this example problem, the principal tensile stress in the joint would have been less than the maximum allowed tensile stress, thus eliminating the need for the special joint reinforcement other than the minimum required reinforcement. However, the practice of increasing footing depth to eliminate the need for the special joint reinforcement should be limited to increasing the footing depth a maximum of 6 inches.
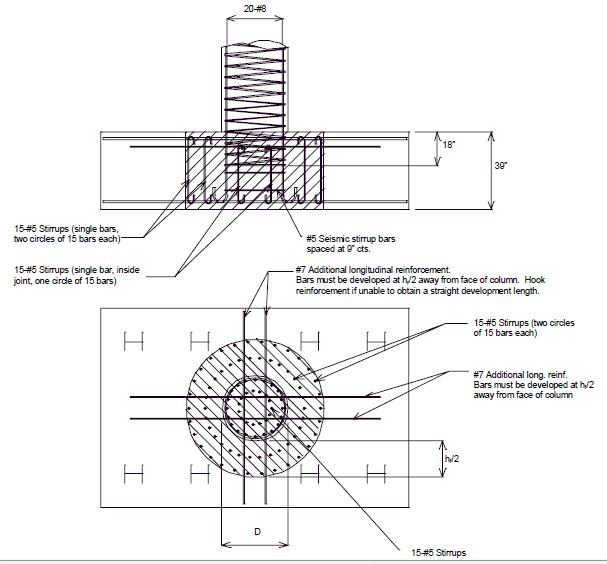
751.11.2.1 Elastomeric Bearings
General
Elastomeric Bearing design shall follow AASHTO LRFD “Method A”.
The elastomeric expansion bearings and fixed bearings for steel girders consist of a sole plate and elastomeric bearing pad. Elastomeric bearings at integral end bents and fixed bearings for prestressed girders at intermediate bents consist of elastomeric bearing pad without a sole plate.
The sole plates are flat or beveled to match the profile grade of the roadway surface along the centerline of the girder. If the profile grade is equal to or less than 0.01 or the total drop across the bearing is equal to or less than 1/8 inch, then a flat sole plate may be used and if necessary, elastomer thickness increased to address the bearing rotation due to the profile grade. Sole plates are used to anchor girders, ensure uniform distribution of the compressive stress and strain over bearing area and reduce dead load bearing rotations.
At fixed bearings, girder chairs may be considered as an alternate if roadway slope, rotation or bearing pressure is requiring tall or large bearing pads.
The elastomer bearing pad shall be 60 durometer hardness and reinforced with 1/8 inch steel shim plates when laminated pads are required by design.
When rectangular bearings are used, increased rotation bearing capacity can be achieved by orientating the pad with the shorter dimension of the pad parallel with the span of the girder.
Plain elastomeric bearing pads should be utilized where vertical loads, translations and rotations are relatively small. For integral end bents, use ½” fixed plain pads when taper due to girder slope or grade is less than 1/8”, or use a laminated bearing pad when taper equals or exceeds 1/8” due to girder slope or grade. In the rare circumstance when intermediate bents are made integral by extending the beam cap stirrups into the diaphragm, consideration can be given to utilizing ½” plain pads under similar conditions of slope.
Laminated elastomeric bearing pads should be utilized where there is greater need for vertical loading, translational and rotational capacity. For non-integral end bents, non-integral intermediate bents and for integral end bents when taper exceeds 1/8” due to girder slope or grade use laminated elastomeric bearing pads where the pad thickness and number of laminates is based on design that should account for larger vertical loads, translation, rotation and meet slope of girder and grade requirements.
Size Limitations
Use the values in the standard bearing pad tables if possible.
Bearing pad length shall be 8” minimum for MoDOT Standard Prestressed (P/S) I-Girders, Adjacent P/S Box Beams and Steel I-Girders.
Bearing pad length shall be 5” minimum for P/S NU Girders and P/S Spread Box Beams. Not preferred but for consideration of some lower bound limits as used by the Nebraska Department of Roads (NDOR) that developed the NU I-Girder, for P/S NU Girders only, and based on NDOR guidelines, a 4” minimum bearing pad length and/or 2 ft. minimum bearing pad width can be used with Structural Project Manager or Structural Liaison Engineer approval.
Plain Fixed for P/S I-Girder:
W, width of bearing ≤ Bottom flange width – 1.5”
Plain Fixed for steel girders:
Bottom flange width – 2” ≤ W ≤ Bottom flange width
Laminated Fixed for P/S I-Girder:
W = Bottom Flange width – 1.5”
Laminated Expansion Bearing Pads for PS I-Girders and
Laminated Fixed and Expansion Pads for Steel Girders:
9” ≤ W ≤ Bottom flange width + 7”
Stability
The following requirement shall be met for ensuring stability of the bearing pad:
Rectangular Pads
Circular Pads
Temperature Movement
Determining temperature movements for bearings requires the calculation of the thermal origin of the bridge. To accomplish this, the stiffness of each bent must be calculated. Once the thermal origin is established, the total temperature movement for each bearing location can be found by the following equations:
(temperature range)x(distance from thermal origin)(0.65)
Where:
= maximum shear deformation of the pad | |
= 1.2 for laminated pads | |
= 1.0 for PTFE bearings | |
= coefficient of thermal expansion | |
= 0.0000065 IN/IN/ºF (steel superstructure) | |
= 0.000006 IN/IN/ºF (concrete superstructure) | |
(0.65) | = 65% reduction due to forgiving nature of elastomer (LRFD 14.7.6.3.4) |
temperature range | = 150ºF (steel superstructure) |
= 120ºF (concrete superstructure) |
Shear Deformation
Both plain elastomeric and laminated elastomeric shall meet the following criteria for shear deformation.
Where:
= total elastomer thickness, in.
The following table represents the available heights of laminated expansion pads that are required due to the shear deformation criteria. PTFE type bearings shall be used if
Laminated Expansion Pads | |||||
---|---|---|---|---|---|
, in. | Interior layer thickness, in. | Exterior layer thickness, in. | Failed to parse (SVG (MathML can be enabled via browser plugin): Invalid response ("Math extension cannot connect to Restbase.") from server "https://wikimedia.org/api/rest_v1/":): {\displaystyle \,h_{rt}} , in. | n | C, in. |
1/2 | 1/2 | 1/4 | 1 | 2 | 1 1/4 |
3/4 | 1/2 | 1/4 | 1 1/2 | 3 | 1 7/8 |
1 | 1/2 | 1/4 | 2 | 4 | 2 1/2 |
1 1/4 | 1/2 | 1/4 | 2 1/2 | 5 | 3 1/8 |
1 1/2 | 1/2 | 1/4 | 3 | 6 | 3 3/4 |
1 3/4 | 1/2 | 1/4 | 3 1/2 | 7 | 4 3/8 |
2 | 1/2 | 1/4 | 4 | 8 | 5 |
2 1/4 | 1/2 | 1/4 | 4 1/2 | 9 | 5 5/8 |
2 1/2 | 1/2 | 1/4 | 5 | 10 | 6 1/4 |
Where:
C | = total thickness of bearing including steel shims, in. |
n | = total number of interior layers of elastomer + 1* |
* The additional “1” is accounting for the two exterior layers as per LRFD 14.7.5.3.5
Compressive Stress
Service loads without including dynamic load allowance shall be used for design checks.
At intermediate bents with 2 bearing pads per girder line (i.e. PS I-girders), use ½ of the live load reaction for each pad.
Total Load
Plain Elastomeric Pad
Laminated Elastomeric Pad
and
Minimum Dead Load
The 200 psi minimum requirement is intended for preventing the horizontal crawling of the bearing when it is not attached to the top surface. This requirement has been applied to the bearing designs detailed in EPG 751 even though these bearings are detailed with positive attachment to the flange of the girder. Compliance with the requirement is desirable but is not mandatory if it results in a special bearing design or special superstructure treatments.
Where:
= compressive stress due to total load = | |
=compressive stress due to maximum dead load = | |
=compressive stress due to minimum dead load = | |
G | = shear modulus = 0.130 ksi for compressive stress calculations |
S | = shape factor for thickest layer of elastomer = |
S | = shape factor for circular pads = |
= thickness of the ith elastomer layer, in. |
Rotation
Rotations shall be taken as the maximum possible change in slope between the top and bottom surfaces of the bearing caused by the initial lack of parallelism between the bottom of girder flange and top of bearing and the girder end rotation due to imposed loads and movements. Rotations may be calculated by a straight-line approximation of dead and live load deflections taken at 1/10 or 1/4 points. The following equation must be satisfied to ensure that uplift does not occur under any combination of loads and corresponding rotation:
Rectangular Laminated Elastomeric Pad
Where:
L | = length of bearing pad in the direction of traffic |
W | = width of bearing pad in the direction perpendicular to traffic |
G | = shear modulus = 0.200 ksi for rotation calculations |
= maximum rotation about the transverse axis due to initial lack of parallelism and total service load, rad | |
n | = total number of interior layers of elastomer + 1* |
* The additional “1” is accounting for the two exterior layers as per LRFD 14.7.5.3.5
Plain elastomeric pads contained within integral concrete diaphragms are not subject to this rotation requirement.
This criteria is intended as an uplift check. If uplift is not possible at the bearing due to an integral diaphragm/beam at the abutment or an integral diaphragm/beam at an intermediate bent, then this criteria would not be applicable.
Circular Laminated Elastomeric Pad
Where:
D = diameter of pad
Compressive Deflection
Deflections of elastomeric bearings should be limited to ensure that deck joints and seals are not damaged. Also, bearings that are too flexible can produce a small step across a deck joint giving rise to a high impact loading when traffic passes from one girder to the other. The maximum relative deflection across a joint is suggested to be less than 1/8”.
Initial compressive deflection of plain elastomeric or in any layer of steel reinforced elastomeric bearing at the service limit state without impact shall not exceed .
Values for compressive strain can be calculated by using LRFD Figure C14.7.6.3.3-1 for 60 durometer reinforced bearings.
There is a code check for compressive deflection of a single layer but no code check for total compressive deflection.
Taper
When the difference between the slope of the girder and the slope of a bearing pad exceeds 1/8” taper shall be considered. For laminated expansion pads for both PS I-Girders and steel girders and for laminated fixed pads for steel girders where sole plates are required, sole plates shall be tapered to account for the girder slope. Sole plates shall have a minimum thickness of 1 1/2” at the centerline of bearing, and a minimum thickness of 1” at the edge. Plain fixed pads shall not be tapered. At integral end bents where girder slope is greater than 1/8”, use laminated fixed pads. Laminated bearing taper is provided by tapering the top shim to match the slope of the girder to the nearest 1/8” total difference in thickness. Thickness of shims shall be a minimum of 1/8” and a maximum of 1/2”. For excessive girder slopes it may be necessary to taper the top two shims with a maximum combined taper of the bearing of 3/4 inch. Tapered layers of elastomer are not permitted.
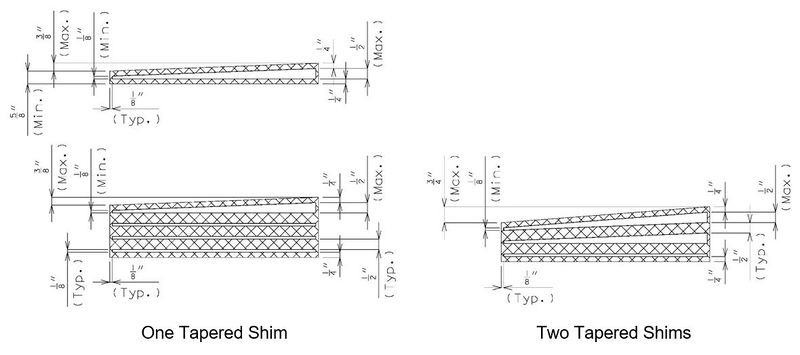
Anchor Bolts
Check with Structural Project Manager or Liaison before using anchor bolts other than ASTM F1554. When anchor bolts are used for laminated fixed for steel girders or laminated expansion for steel and P/S I-girders, they should be designed for a minimum horizontal force equal to 25% of the maximum dead load applied to the bearing. With SPM approval for rehab superstructure job designer may design anchor bolt for a minimum horizontal force equal to 15% of the maximum dead load applied to the bearing. Designer may ignore live load in horizontal force computation. Anchor bolts shall be ASTM F1554 Grade 55 unless higher grade anchor bolts are required to meet design requirements. Grade 105 bolts shall not be used in applications where welding to the bolt is required. (Revise anchor bolt notes in EPG 751.50 Standard Detailing Notes for plans with different grade and nuts, e.g. “ASTM F1554 Grade 55” to “ASTM F1554 Grade 105” and “ASTM A563 Grade A Heavy Hex nuts” to “ASTM A563 Grade DH Heavy Hex nuts”.)
Bolt properties (Updated in 2022) | ||||||||||
---|---|---|---|---|---|---|---|---|---|---|
Bolt Type | Nominal Bolt Diameter (in.) |
Min. Tensile Strength (ksi) |
Min. Yield Strength (ksi) |
Comments | ||||||
ASTM F1554 (Grade 36) | ½” to 4” | 58 | 36 | Preferred specification for structural supports anchored into concrete. | ||||||
ASTM F1554 (Grade 55) | ½” to 4” | 75 | 55 | |||||||
ASTM F1554 (Grade 105) | ½” to 3” | 125 | 105 | |||||||
ASTM A449 Type 1 Type 3 (weathering) |
¼” to 1” | 120 | 92 | Material properties are applicable for old A325 bolts (Pre-2016). May be manufactured as a threaded rod. | ||||||
over 1” to 1 ½” | 105 | 81 | ||||||||
1 ¾” to 3” | 90 | 58 | Typically used for larger diameter headed anchors for bearings in girder shelves. | |||||||
ASTM A307 | ¼” to 4” | 60 | NA | Typically used for regular strength steel connections. Should not be used for applications that require significant tensile or flexural forces on the bolt. | ||||||
ASTM F3125 Grade A325 Type 1 Type 3 (weathering) |
½” to 1 ½” | 120 | 92 | Typically used as high strength fasteners, but also used as headed anchors for bearings in girder shelves. | ||||||
ASTM F3125 Grade A490 Type 1 Type 3 (weathering) |
½” to 1 ½” | 150 | 130 | Typically used as high-strength fastener. Galvanization is not allowed. | ||||||
Note: The above table is a comprehensive list for bolts typically used in structural applications on MoDOT projects. These values will aid designers when substitutions need to be made for similar design applications. |
Anchor bolts are used on bearings with sole plates. For bridges that require seismic details only, design anchor bolts for flexure and shear induced from horizontal seismic forces and design for tension due to uplift forces if present. For bridges that require a seismic analysis, design anchor bolts for flexure induced from horizontal seismic forces and separately design for the effects of combined tension and shear.
Limit the number of bolts per bearing to four. Concrete Shear blocks shall be used when anchor bolts cannot be designed to resist earthquake loading. For shear blocks details for P/S girder see, EPG 751.22.3.13 Concrete Shear Blocks. Use similar details for shear blocks for steel girder.
Bearing details are shown in EPG 751.11.3 Details for two 1 ½”, 2” and 2 ½“ diameter anchor bolts. Consult Structural Project Manager before using bolt diameters larger than 2 1/2". Revise bearing details if four anchor bolts are required by design. For larger than 2 ½” diameter anchor bolt revise details in EPG 751.11.3.5 Anchor Bolts, bearing details and anchor bolt notes in EPG 751.50 Standard Detailing Notes.
For seismic details only (strength limit states)
Anchorage shall be adequate to resist lateral loads.
Horizontal factored shear force, in kips per girder
For expansion bearings, transverse FT = max (As, 0.25)(DL) per girder & longitudinal FL = 0.
Where DL = unfactored dead load reaction at the bent, kips
- As = Acceleration Coefficient (effective peak ground acceleration coefficient)
For fixed bearings, transverse FT = max (As, 0.25)(DL) per girder and Longitudinal FL = max (As, 0.25)(segment weight)/(# of girders)
Segment weight includes the full width of superstructure and should be distributed appropriately among fixed bents.
When centrifugal forces are present, they should be checked independently (not combined with seismic loads shown above or below). Use a 1.75 load factor with the centrifugal force and check resistance at the Strength Limit state as described below.
For complete seismic analysis
Anchor bolt designs must meet requirements for strength limit states from above as well as seismic forces from seismic analysis. Anchorage shall be adequate to resist lateral loads as well as uplift force from seismic analysis.
At Intermediate bent,
where: FH = horizontal seismic force per girder, kips
- If columns are designed for plastic hinging, use the plastic hinging shear.
= summation of top of column longitudinal shears at the bent
= summation of top of column transverse shears at the bent
NG = number of girders at the bent
At end bents
Use the same formula as above, except substitute the abutment shears in place of the top of column shears.
Pu = horizontal factored shear force per bolt, kips
nb = the number of bolts per girder
For intermediate bent, R = 1.0
For end bent, R = 1.0 for seismic category A & B and 0.8 for seismic category C & D.
Flexural Resistance
Factored flexural stress shall be less than or equal to the nominal flexural resistance. Assume the bolt is restrained from rotation by the sole plate, but free to translate.
M = Pu x L/2 = maximum moment per bolt, inch-kips
L = moment arm from center of sole plate to top of the beam cap, inches
S = section modulus for the bolt , cubic inches.
D = minimum body diameter, inches. For F1554 bolts use D = 0.92 x nominal bolt diameter. Alternately, the minimum body diameter can be retrieved from ASTM F1554 Table 4. For all other bolt types the nominal bolt diameter may be used because the bolt is unthreaded in the flexural zone and the minimum body diameter is similar to the nominal diameter.
Where:
∅f = 1.0 resistance factor for seismic details only (strength limit states) and for complete seismic analysis
Yield strength of the anchor bolt, FY = 55 ksi for Grade 55 and FY = 105 ksi for Grade 105
Shear Resistance
Factored shear force shall be less than or equal to the nominal shear resistance.
where:
∅s = 0.75 resistance for seismic details only (strength limit states) and 1.0 for complete seismic analysis
Nominal shear resistance of an F1554 anchor bolt, Rn = 0.5AbFubNs LRFD 6.13.2.12-1, C6.13.2.7 and 14.8.3
Note: ASTM F1554 allows the body diameter of the bolt to be reduced to provide an area not less than the stress area of the threaded portion of the bolt. For this reason, there are no differences in calculation for threads beings included or excluded from the shear plane. If another type of bolt is used for any reason the nominal shear resistance should be determined from LRFD Eq. 6.13.2.7-1 or 2.
Ab = nominal area of the anchor bolt, square inches
Fub = minimum tensile strength of the anchor bolt, ksi
- = 75 ksi for Grade 55, 125 ksi for Grade 105
Ns = number of shear plane per anchor bolt = 1
D = nominal bolt diameter, inches
Tensile Resistance
Factored tensile force shall be less than or equal to the nominal tensile resistance.
T = the maximum seismic tensile (uplift) force (DL ± EQ) per girder from the seismic analysis, kips. If (DL+EQ) and (DL-EQ) are both compressive, then there is no need to design the bolts for tensile force.
Where:
∅t = 0.8 resistance factor for seismic details only (strength limit states) and 1.0 for complete seismic analysis
nb = the number of bolts per girder
Nominal tensile resistance of the anchor bolt, Tn = 0.76AbFub LRFD 6.13.2.10.2-1 and 14.8.3
- where:
- Ab = nominal area of the anchor bolt, square inches
- Fub = minimum tensile strength of the anchor bolt, ksi
- = 75 ksi for Grade 55, 125 ksi for Grade 105
Combined Tension and Shear Resistance
The resistance of anchor bolts for combined tension and shear force shall be determined in accordance with LRFD 6.13.2.11.
If , then Tn = 0.76AbFub LRFD 6.13.2.11-1
Otherwise
- LRFD 6.13.2.11-2
Strength Limit States and Seismic Details only
Maximum horizontal factored shear force reaction, FH for given anchor bolts and total thickness of bearing including steel shims:
Max. shear deformation of the pad, ∆s, in. | 1/4 | 1/2 | 3/4 | 1 | 1 1/4 | 1 1/2 | 1 3/4 | 2 | 2 1/4 | ||
---|---|---|---|---|---|---|---|---|---|---|---|
Total thick. of bearing including steel shims, C in. | 1 1/4 | 1 7/8 | 2 1/2 | 3 1/8 | 3 3/4 | 4 3/8 | 5 | 5 5/8 | 6 1/4 | ||
Anchor Bolt Type | No. of Anchor Bolt |
Nominal dia. of anchor bolt, in. | Maximum horizontal factored shear force reaction, FH | ||||||||
ASTM F1554 Grade 55 |
2 | 1.5 | 28 | 22 | 17 | 15 | 13 | 11 | 10 | 9 | 8 |
2 | 67 | 51 | 41 | 35 | 30 | 26 | 23 | 21 | 19 | ||
2.5 | 131 | 100 | 81 | 68 | 58 | 51 | 46 | 41 | 38 | ||
4 | 1.5 | 57 | 43 | 35 | 29 | 25 | 22 | 20 | 18 | 16 | |
2 | 135 | 103 | 83 | 69 | 60 | 53 | 47 | 42 | 38 | ||
2.5 | 263 | 200 | 162 | 136 | 117 | 103 | 91 | 82 | 75 | ||
ASTM F1554 Grade 105 |
2 | 1.5 | 54 | 41 | 33 | 28 | 24 | 21 | 19 | 17 | 15 |
2 | 128 | 98 | 79 | 66 | 57 | 50 | 45 | 40 | 37 | ||
2.5 | 251 | 191 | 154 | 129 | 111 | 98 | 87 | 79 | 72 | ||
4 | 1.5 | 108 | 83 | 67 | 56 | 48 | 42 | 38 | 34 | 31 | |
2 | 257 | 196 | 158 | 133 | 114 | 100 | 89 | 81 | 73 | ||
2.5 | 502 | 382 | 309 | 259 | 223 | 196 | 174 | 157 | 143 | ||
Notes: | Calculations were based on 1-1/2" thick sole plate at centerline of bearing. Flexural resistance calculations were based on minimum body diameter of the anchor bolt. For centrifugal forces, check anchor bolts for separate load. For complete seismic analysis case, design anchor bolts as explained above. |
751.22.2.7 Dowel Bars
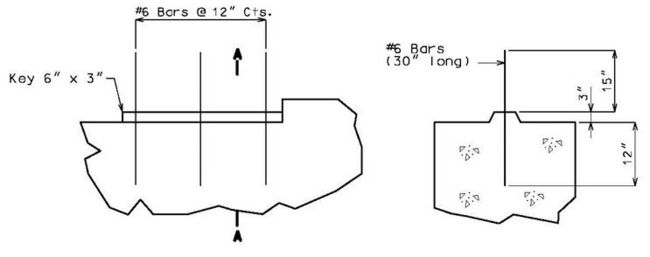
PART ELEVATION (FIXED BENT) |
SECTION A-A |
- Dowel bars shall be used for all fixed intermediate bents under prestressed superstructures. Generally, for typical bridges that require seismic details only (strength limit states), shear resistance from shear key is not considered.
Dowel bars connect standard concrete diaphragms and beams on concrete girder bridges (standard fixed diaphragms are those with beam stirrups NOT extending up into the diaphragm). For a calculated seismic vertical reaction or an anticipated foundation settlement resulting in a net tensile reaction, use the development length of dowel bars into beam and into diaphragm based on dowel bar size. If the dowel bars are not exposed to net tension a 15-inch embedment shall be used regardless of bar size. Dowel bars size and spacing shall be determined by shear design of the bars. (Minimum #6 Bars @ 12" cts.). Dowel bars should be designed for a minimum horizontal force equal to 25% of the maximum dead load applied to the bearing. Live load is ignored in horizontal force computation. For seismic design category SDC B, C and D, dowel bars shall develop minimum Ld into diaphragm but shall not extend into slab and develop minimum Ld into beam but 3” minimum clear from bottom face of the beam. Dowel bars shall not be hooked to meet development requirements.
- The number of dowels must also fit into the space available on the key:
- min. bar size = #6; max. bar size = #11
- min. spacing = 6"; max. spacing = 12"
- min. end distance = 3"; max. end distance = 6" (≤ half the spacing)
For seismic details only (strength limit states)
Horizontal factored shear force, in kips
For expansion bearings, transverse FT = max (As, 0.25)(DL) & longitudinal FL = 0 per girder.
- Where DL = unfactored dead load reaction at the bent, kips
- As = Acceleration Coefficient (effective peak ground acceleration coefficient)
For fixed bearings, Transverse FT = max (As, 0.25)(DL) and Longitudinal FL = max (As, 0.25)(segment weight) at bent.
- Segment weight includes the full width of superstructure and should be distributed appropriately among fixed bents.
For complete seismic analysis
Dowel bar designs must meet requirements for strength limit states from above as well as seismic force demand from seismic analysis.
- At Intermediate bent,
- where:
- FH = horizontal seismic force per bent, kips
- If columns are designed for plastic hinging, use the plastic hinging shear.
- ∑VL = summation of top of column longitudinal shears at the bent
- ∑VT = summation of top of column transverse shears at the bent
- Pu = Horizontal factored shear force per dowel bar, kips
- nd = number of dowel bars
Shear Resistance
Factored shear force shall be less than or equal to the nominal shear resistance.
- Pu ≤ ∅s x Rn
- where:
- ∅s = 0.75 resistance for seismic details only (strength limit states) and 1.0 for complete seismic analysis
- Nominal shear resistance of the dowel bar, Rn = 0.625 AbFub, kips
- Note: Since there is no reduced areas as seen in bolts and there is no reduction for bolted connection length, use 0.625 instead of 0.5.
- Ab = = area of the dowel bar, square inches
- Fub = minimum tensile strength of the dowel bar, ksi
- Fub = 80 ksi for Grade 60
- D = diameter of the dowel bar, inch
Tensile Resistance
Factored tensile force shall be less than or equal to the nominal tensile resistance.
- T = the maximum seismic tensile (uplift) force (DL ± EQ) from the seismic analysis, kips. If (DL+EQ) and (DL-EQ) are both compressive, then there is no need to design the dowel for tensile force.
- where:
- ∅t= 0.8 resistance factor for seismic details only (strength limit states) and 1.0 for complete seismic analysis
- nd = the number of dowel bars
- Nominal tensile resistance of the dowel bar, Tn = AbFub Kips
- Note: Since there is no pretension or reduced areas as seen for bolts, the 0.76 factor is not warranted.
- Ab = area of the dowel bar, square inches
- Fub = minimum tensile strength of the dowel bar, ksi
- Fub = 80 ksi for Grade 60
Combined Tension and Shear Resistance
The resistance of dowel bars for combined tension and shear force shall be determined in accordance with LRFD 6.13.2.11.
- Note: Since there is no pretension or reduced areas as seen for bolts, the 0.76 factor is not warranted.
- If , then Tn = AbFub
- Otherwise
- Otherwise
751.31.1.2 Rigid Frame- No Tie or Web Beam
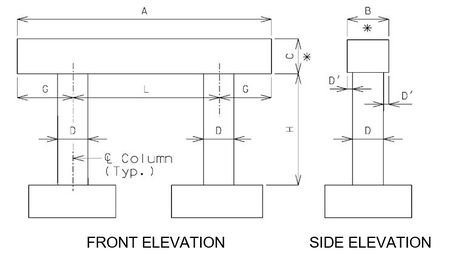
Beam
- A = Length to be determined by the superstructure requirements or the minimum support length required for earthquake criteria, to the
- nearest 1”. Use square ends.
- B = Width to be determined by the minimum of: superstructure requirements, minimum support length required for earthquake criteria, or
- column diameter + 6”. (6” increments) (*)
- C = Depth as required by design. 2’-6” minimum and no less than the column diameter. (3” increments) (*)
- * For SDC A ratio of beam width to beam depth, B/C, shall be ≤ 1.25. For SDC B, C and D, beam depth shall be proportioned to D ≤ C ≤ 1.25 D. SGS 8.13.5-1
Columns
- D = Column diameter. 2’-6” minimum. Use 3’-0” minimum when the beam depth exceeds 3’-6”. (6” increments)
- D' = Beam width overhang. Controlled by one of the following:
- 1) Beam width controlled by superstructure requirements
- 3” ≤ D' ≤ 6”
- 2) Beam width controlled by minimum support length required for earthquake criteria.
- 3” ≤ D' ≤ 15”
- 2) Beam width controlled by minimum support length required for earthquake criteria.
- L = Spacing as determined by design, with no limit. (1” increments)
- G = Overhang as determined by design, with no limits.
- H = Column height as required by grade and footing elevations. Use construction joint in column when H exceeds 35’-0”.
- NOTE: Try to keep columns and beams the same size where possible for economy of construction.
751.31.1.3 Web Beam – Web Supporting Beam
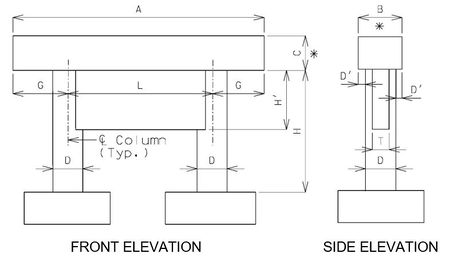
Beam
- A = Length to be determined by the superstructure requirements or the minimum support length required for earthquake criteria, to the
- nearest 1”. Use square ends.
- B = Width to be determined by the minimum of: superstructure requirements, minimum support length required for earthquake criteria, or
- column diameter + 6”. (6” increments) (*)
- C = Depth as required by design. 2’-6” minimum and no less than the column diameter. (3” increments) (*)
- * For SDC A ratio of beam width to beam depth, B/C, shall be ≤ 1.25. For SDC B, C and D, beam depth shall be proportioned to D ≤ C ≤ 1.25 D. SGS 8.13.5-1
Columns
- D = Column diameter. 3’-0” minimum. (6” increments)
- D' = Beam width overhang. Controlled by one of the following:
- 1) Beam width controlled by superstructure requirements
- 3” ≤ D' ≤ 6”
- 2) Beam width controlled by minimum support length required for earthquake criteria.
- 3” ≤ D' ≤ 15”
- 2) Beam width controlled by minimum support length required for earthquake criteria.
- L = Spacing as determined by design, with 35'-0" maximum. (1” increments)
- G = Overhang as determined by design, with no limits.
- H = Column height as required by grade and footing elevations.
Webs
- T = Web thickness. For a 3’-0” column diameter, use T = column diameter. For column diameters ≥ 3’-6”, use T = 0.5 x (column diameter).
- H' = See bottom elevations of web given on the Design Layout.
- NOTE: Try to keep columns and beams the same size where possible for economy of construction.
751.31.1.4 Tie Beam
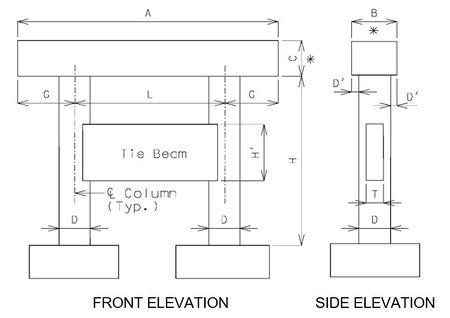
Beam
- A = Length to be determined by the superstructure requirements or the minimum support length required for earthquake criteria, to the
- nearest 1”. Use square ends.
- B = Width to be determined by the minimum of: superstructure requirements, minimum support length required for earthquake criteria, or
- column diameter + 6”. (6” increments) (*)
- C = Depth as required by design. 2’-6” minimum and no less than the column diameter. (3” increments) (*)
- * For SDC A ratio of beam width to beam depth, B/C, shall be ≤ 1.25. For SDC B, C and D, beam depth shall be proportioned to D ≤ C ≤ 1.25 D. SGS 8.13.5-1
Columns
- D = Column diameter. 3’-0” minimum. (6” increments)
- D' = Beam width overhang. Controlled by one of the following:
- 1) Beam width controlled by superstructure requirements
- 3” ≤ D' ≤ 6”
- 2) Beam width controlled by minimum support length required for earthquake criteria.
- 3” ≤ D' ≤ 15”
- 2) Beam width controlled by minimum support length required for earthquake criteria.
- L = Spacing as determined by design, with 30'-0" maximum. (1” increments)
- G = Overhang as determined by design, with no limits.
- H = Column height as required by grade and footing elevations.
Tie Beam
- T = Tie beam thickness. Minimum T = 0.5 x (column diameter).
- H' = See bottom elevations of tie beam given on the Design Layout. Minimum H' = 2 x T (round to the next foot higher).
- NOTE: Try to keep columns and beams the same size where possible for economy of construction.
751.31.1.5 Tie Beam with Change in Column Diameter
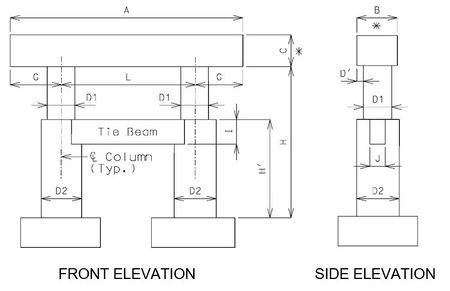
Beam
- A = Length to be determined by the superstructure requirements or the minimum support length required for earthquake criteria, to the
- nearest 1”. Use square ends.
- B = Width to be determined by the minimum of: superstructure requirements, minimum support length required for earthquake criteria, or
- column diameter + 6”. (6” increments) (*)
- C = Depth as required by design. 2’-6” minimum and no less than the column diameter. (3” increments) (*)
- * For SDC A ratio of beam width to beam depth, B/C, shall be ≤ 1.25. For SDC B, C and D, beam depth shall be proportioned to D1 ≤ C ≤ 1.25 D1. SGS 8.13.5-1
Columns
- D1 = Column diameter. 3’-0” minimum. (6” increments)
- D2 = Column diameter, Minimum of (D1 + 6”). Check lap of vertical reinforcement required. See Structural Project Manager.
- D' = Beam width overhang. Controlled by one of the following:
- 1) Beam width controlled by superstructure requirements
- 3” ≤ D' ≤ 6”
- 2) Beam width controlled by minimum support length required for earthquake criteria.
- 3” ≤ D' ≤ 15”
- 2) Beam width controlled by minimum support length required for earthquake criteria.
- L = Spacing as determined by design, with a 30’-0” maximum with tie beams and no limit without tie beams. (1” increments)
- G = Overhang as determined by design, with no limits.
- H = Column height as required by grade and footing elevations.
- H' = Approximately 0.5 x H. Top of tie beam should be at the same elevation as the top of the larger diameter columns in order to
- minimize the number of construction joints. Top of tie beam may be located on the Design Layout.
Tie Beam
- I = Depth as required by design. Minimum of 3’-0” (3” increments).
- J = Width as required by design. Minimum of (0.5 x D1).
- NOTE: Try to keep columns and beams the same size where possible for economy of construction.
751.31.2.3 General Design Assumptions
* Use only if specified on the Design Layout or as stated by the guidelines in this article.
** For column spacings greater than 30'-0", tie beams are not to be used, unless the web supports the beam.
Elevations for General Intermediate Bent
General
- The following are general design guidelines for the design of intermediate bents.
- Rigid frame design is to be used for designing Intermediate Bents and Piers.
- The joint between the beam and column, and web or tie beam and column, shall be assumed to be integral for all phases of design and must be analyzed for reinforcement requirements as a “Rigid Frame”.
- The joint between the column and footing is assumed to be “fixed”, unless foundation flexibility needs to be considered as required by the Structural Project Manager.
Beam
- Beams shall be designed for vertical loads, including a dynamic load allowance (impact) and components of horizontal forces.
- The gross concrete section, without contribution from reinforcement, shall not rupture under service dead loads. In addition, longitudinal reinforcement shall be distributed to control cracking at the Service-I limit state.
- Fatigue design should not control the size of reinforcement in the beam. LRFD 5.5.3.2 may be ignored for open concrete intermediate bents.
- The minimum reinforcement shall be such that the factored flexural resistance, Mr, is greater than or equal to the lesser of:
- Minimum Tensile Reinforcement
- The amount of tensile reinforcement shall be adequate to develop a factored flexural resistance, Mr, at least equal to the lesser of either:
- 1) Mcr = cracking moment LRFD Eq. 5.7.3.3.2-1
- 2) 1.33 times the factored moment required by the applicable strength load combinations specified in LRFD Table 3.4.1-1.
- Additional reinforcement is required in the sides of the beam. The following table gives adequate steel for both temperature and shrinkage (LRFD 5.10.8), and skin reinforcement (LRFD 5.7.3.4).
Beam Height, H | Number – Bar Size |
H ≤ 36” | 4 - #6 |
36” < H < 54” | 5 - #6 |
54” ≤ H ≤ 72” | 6 - #6 |
H > 72” | By Design (LRFD 5.7.3.4) |
Tie Beam
- Use a tie beam when specified on the Design Layout or by the Structural Project Manager or when the unsupported height exceeds 30 feet, except as noted
- Do not use tie beams on grade separations.
- Do not use tie beams when column spacing exceeds 30 feet. For this situation, use a minimum column diameter of in lieu of a tie beam.
- Additional side reinforcement shall be designed for temperature and shrinkage (LRFD 5.10.8), and skin reinforcement (LRFD 5.7.3.4).
Unsupported Height
- The unsupported height is the distance from the bottom of the beam to the top of the footing. If the distance from the ground line to the top of footing is 10 feet, the unsupported height and the fixed point may be measured from the bottom of the beam to the ground line plus 1/2 the distance from the ground line to the top of the footing.
- For single column intermediate bents, the column shall be considered “fixed” at the top of footing for all conditions.
Columns
- Use round columns for all bridges, unless otherwise specified on the Design Layout.
- Tops of column shall be designed for vertical loads with consideration of dynamic load allowance (impact) and maximum components of horizontal forces. Bottom of columns do not require impact forces to be included.
- The minimum area of reinforcement, As, shall be taken as the greater of:
- LRFD 5.7.4.2
- Where:
- = gross area of section. (in.²)
- For typical columns with f’c = 3 ksi, the 1% of column gross area will control. MoDOT prefers to follow ACI 10.9 and recognize LRFD 5.7.4.2 when it would control. (The minimum area of reinforcement based on LRFD is significantly less than ACI for f’c = 3 ksi).
Minimum Allowable Bars for Column Reinforcement Design Column Diameter Vertical Reinforcement
(Assuming 1% of Column Gross Area)2’-6” 9 - #8 3’-0” 13 - #8 3’-6” 18 - #8 4’-0” 23 - #8 4’-6” 29 - #8 5’-0” 29 - #9 5’-6” 35 - #9 6’-0” 41 - #9
- The maximum reinforcement shall be limited by the following requirements:
SGS 8.8.1
- (Preferred max for seismic design.)
- (Absolute max, LRFD 5.7.4.2)
- Spacing limitations given in this article.
Maximum Allowable Number of Bars for the Given Bar Sizes Column Diameter Maximum Number of Bars #8 #9 #10 #11 2’-6” 18 18 17 15 3’-0” 22 22 21 18 3’-6” 26 26 26 22 4’-0” 30 30 30 25 4’-6” 34 34 34 29 5’-0” 38 38 32 5’-6” 43 42 36 6’-0” 47 46 40
- Above table is applicable for standard dowel bar arrangments, see EPG 751.31.3.2.
- A preliminary economic analysis should be conducted before determining the number of columns and column spacing. For the analysis, assume the rates for Concrete, Class 1 and Class 2 Excavation, and Piles. Omit reinforcing bars in the cost analysis.
Column Spacing
- Columns, with the exception of web supporting beam type bents, shall be spaced, to the nearest 1”, in which balanced positive and negative beam moments are produced. A positive beam moment up to 10% larger than the negative beam moment is acceptable. Strength Limit State Load Combinations shall be used to determine column spacing.
- To estimate centerline-to-centerline spacing for a two column bent, use 72% of the distance from centerline of outside girder to centerline of outside girder. For a three column bent, use 44% of the centerline-to-centerline distance of outside girders.
Footings
- Footings shall be designed for vertical loads and maximum normal and parallel components of the horizontal forces.
Elevations for Intermediate Bent with Web Beam
Web Supporting Beam
- In analysis, web beams shall be modeled as plate elements. If the ability to model a web beam as a plate element is unavailable, the following may be considered:
Simplified Model
- The web itself is made up of several tie beams (typically 4 tie beams). The moment of inertia of an individual tie beam is equal to the moment of inertia of the web in the bent’s out-of-plane direction divided by the total number of tie beams.
- Any column segment which is connected to the web is treated as a prismatic member with moment of inertia in the bent’s out-of-plane direction equal to the actual column moment of inertia in that direction, and with the moment of inertia in the bent’s in-plane direction equal to the total moment of inertia of web in the bent’s in-plane direction divided by the total number of columns plus the moment of inertia of the column itself. The equivalent column diameter is assumed to be .
Section Views for an Intermediate Bent with Web Beam
In the above example, the moment of inertia of the column in the bent’s in-plane and out-of-plane directions can be calculated as:
Out-of-plane-> | |
In-plane-> |
The equivalent column diameter is then assumed to be .
Thus, the column can be treated as a telescoping column and then the moment magnifier or P-δ slenderness effects can be calculated.
Since the web is made up of 4 tie beams, the moment of inertia of the tie beams in the bent’s out-of-plane direction is:
Reinforcement
- Additional side reinforcement shall be designed for temperature and shrinkage (LRFD 5.10.8), and skin reinforcement (LRFD 5.7.3.4).
Column Spacing
- Columns shall be spaced so that the negative moment in the beam over the outside columns requires a minimum beam depth of 3.0 FT. No attempt should be made to use a column spacing that produces equal positive and negative beam moments. The negative moment is to be determined at the face of the column (for round columns, check moment at the face of an equivalent area square column).
* Use a tie beam if specified on the Design Layout or if the design calls for one.
** For column spacing > 30’, tie beams are not to be used unless the web supports the beam
Elevations for Intermediate Bent with Tie Beam
Change in Column Diameter
- Use rigid frame design.
- If H’ ≤ 0.5H and no tie beam is used, the design may be done assuming the entire column to have the smaller diameter. This will result in a very small error.
Columns
- Use round columns for all bridges, unless otherwise specified on the Design Layout.
- Use two or more columns, as required for the more economical design.
Column Spacing
- Column spacing (to the nearest 1”) should be that which produces balanced positive and negative moments. A positive beam moment up to 10% larger than the negative beam moment is acceptable. Strength Limit State Load Combinations shall be used to determine column spacing.
Reinforcement
- Reinforcement in the beams, column and tie beams for the moments at the joints shall be based on the moment at the face of the column, beam, or tie beam (equivalent square, based on areas, for round columns).
Elevations for Hammer Head Intermediate Bent
Hammer Head Type Intermediate Bent
- Hammer Head type intermediate bents shall be designed according to the applicable provisions listed under the design assumptions for the General intermediate bent guidelines except as follows:
Reinforcement
- Additional side reinforcement shall be designed for temperature and shrinkage (LRFD 5.10.8), and skin reinforcement (LRFD 5.7.3.4).
751.31.2.4 Column Analysis
Refer to this article to check slenderness effects in column and the moment magnifier method of column design. See Structural Project Manager for use of P Delta Analysis.
Transverse Reinforcement
Seismic Design Category (SDC) A
- Columns shall be analyzed as “Tied Columns”. Unless excessive reinforcement is required, in which case spirals shall be used.
Bi-Axial Bending
Use the resultant of longitudinal and transverse moments.
Slenderness effects in Columns
The slenderness effects shall be considered when:
Where:
= unsupported length of column
= radius of gyration of column cross section
= effective length factor
Effects should be investigated by using either the rigorous P-∆ analysis or the Moment Magnifier Method with consideration of bracing and non-bracing effects. Use of the moment magnifier method is limited to members with Klu/r ≤ 100, or the diameter of a round column must be ≥ Klu/25. A maximum value of 2.5 for moment magnifier is desirable for efficiency of design. Increase column diameter to reduce the magnifier, if necessary.
When a compression member is subjected to bending in both principal directions, the effects of slenderness should be considered in each direction independently. Instead of calculating two moment magnifiers, db and ds, and performing two analyses for M2b and M2s as described in LRFD 4.5.3.2.2b, the following conservative, simplified moment magnification method in which only a moment magnifier due to sidesway, δs, analysis is required:
General Procedure for Bending in a Principal Direction
- Mc = δsM2
- Where:
- Mc = Magnified column moment about the axis under investigation.
- M2 = value of larger column moment about the axis under investigation due to LRFD Load Combinations.
- δs = moment magnification factor for sidesway about the axis under investigation
Where:
= | summation of individual column factored axial loads for a specific Load Combination (kip) | |
= | stiffness reduction factor for concrete = 0.75 | |
= | summation of individual column Euler buckling loads |
Where:
= effective length factor = 1.2 min. (see the following figure showing boundary conditions for columns)
= unsupported lenth of column (in.)
Where:
= concrete modulus of elasticity as defined in EPG 751.31.1.1 (ksi)
= moment of inertia of gross concrete section about the axis under investigation
= ratio of maximum factored permanent load moments to maximum factored total load moment: always positive
Column Moment Parallel to Bent In-Plane Direction
= top of footing to top of beam cap
Column Moment Normal to Bent In-Plane Direction
= top of footing to bottom of beam cap or tie beam and/or top of tie beam to bottom of beam cap
Out-of-plane bending Non-integral Bent |
![]() |
Out-of-plane bending Integral Bent |
In-plane bending | ![]() |
Boundary Conditions for Columns
For telescoping columns, the equivalent moment of inertia, I, and equivalent effective length factor, K, can be estimated as follows:
Telescoping Columns
Where:
= length of column segment
= moment of inertia of column segment
= total length of telescoping column
Equivalent Effective Length Factor
Where:
= modulus of elasticity of column
= equivalent moment of inertia of column
= total length of telescoping column
=elastic buckling load solved from the equations given by the following boundary conditions:
Fixed- Fixed Condition
Hinged-Fixed Condition
Where:
and are defined in the previous equations.
Fixed-Fixed with Lateral Movement Condition
Where:
and are defined in the previous equations.
Fixed-Free with Lateral Movement Condition
Where:
and are defined in the previous equations.
751.31.3 ReinforcementFor Seismic detail requirements for seismic design category, SDC B, C and D, See EPG 751.9.1.2 LRFD Seismic Details. 751.31.3.2 Column
751.37.1.6 Drilled Shaft General Detail ConsiderationsFor Seismic detail requirements for seismic design category, SDC B, C and D, See EPG 751.9.1.2 LRFD Seismic Details. ![]() Pay items shown in above table are for example only, show actual pay items and quantities in plan details for specific project. Notes:
751.37.6.1 Reinforcement DesignDrilled shaft structural resistance shall be designed similarly to reinforced concrete columns. The Strength Limit State and applicable Extreme Event Limit State load combinations shall be used in the reinforcement design. Longitudinal reinforcing steel shall extend below the point of fixity of the drilled shaft at least 10 ft. in accordance with LRFD 10.8.3.9.3 or the required bar development length whichever is larger. If permanent casing is used, and the shell consists of smooth pipe greater than 0.12 in. thick, it may be considered load carrying. An 1/8" shall be subtracted off of the shell thickness to account for corrosion. Casing could also be corrugated metal pipe. If casing is assumed to contribute to the structural resistance, the plans should indicate the minimum thickness and type of casing required. Minimum clear spacing between longitudinal bars as well as between transverse bars shall not be less than five times the maximum aggregate size or 5 in. (LRFD 10.8.3.9.3). For minimum concrete cover for drilled shaft, see Sec 701.4.12.1. If drilled shaft diameter does not match Sec 701.4.12.1 then use concrete cover for the next greater diameter drilled shaft. For rock sockets use 3” min. clear cover. For longitudinal reinforcement, splicing shall be in accordance with LRFD 5.10.8.4. For transverse reinforcement, lap splices for closed circular stirrups/ties shall be provided and staggered in accordance with LRFD 5.10.4.3. Lap length of 1.3 ld (Class B) for closed stirrups/ties shall be provided in accordance with LRFD 5.10.8.2.6d. For lap length, see EPG 751.5.9.2.8.1 Development and Lap Splice General.
751.37.6.2 Longitudinal ReinforcementLongitudinal reinforcement shall be designed to resist bending in the shaft due to lateral loads. The cross-sectional area for longitudinal reinforcement shall fall within the following limits:
where:
MoDOT prefers to follow LRFD 5.7.4.2 for drilled shafts since for typical cases, the potential exists for load transfer between the concrete and steel casing. (The minimum area of reinforcement based on LRFD is 10 percent less than ACI for f’c = 4 ksi).
751.37.6.4 Transverse ReinforcementMinimum transverse reinforcement shall be designed to resist the potential of diagonal cracking and improve ductility, and to control the stability of the reinforcement cage. Follow the four-step procedure, below for seismic design category SDC A. For Seismic detail requirements for seismic design category, SDC B, C and D, See EPG 751.9.1.2 LRFD Seismic Details. No. 1. Determine if Transverse Reinforcement is Required for Loading
No. 4. Determine Maximum Transverse Shaft Reinforcement Spacing at the Anchorage of Column Reinforcement:
751.38.8.3.1 Spread Footing Reinforcement
751.39.1 DimensionsLong, narrow footings (length to width ratio ≥ 2.0) supporting individual columns are not desirable, and care should be taken to avoid their use unless space constraints or eccentric loading dictate otherwise.
* The maximum pile spacing is 4'-0". ** 3'-0" (Min.) & 6'-0" (Max.) for steel HP piles, 14" CIP piles. 3D (Min.) and 6D (Max.) for 16”, 20" and 24" CIP piles. (D = pile diameter)
751.39.5 ReinforcementUnreinforced Footing - Use only in Seismic Design Category A The term “unreinforced footing” is used for footings where only #6 hairpin bars are required. Do not use unreinforced footing if SDC A, SD1 ≥ 0.1 for applicable routes per Bridge Seismic Design Flowchart. Unreinforced footings shall only be used when the shear line for all piles is within the column projected, or where additional flexural steel is not required by design (not typical).
* See EPG 751.5.9.2.8.2. Notes: Use Class B lap splice of deformed bars in tension.
If SDC A, SD1 ≥ 0.1, provide seismic details similar to SDC B for applicable routes per Bridge Seismic Design Flowchart.
* See See EPG 751.5.9.2.8.2. Notes: Use Class B lap splice of deformed bars in tension.
For anchorage of piles for seismic details for SDC B, C and D, see EPG 751.36.4 Anchorage of Piles for Seismic Details. * For reinforcement in top of the footing and bottom of footing, See EPG 751.5.9.2.8.2 for lap splice.
**Place the top reinforcement uniformly outside the column reinforcement. Use same area of steel in the top of the footing as is required for the bottom. For pile footing joint shear reinforcement requirement for SDC C and D, see EPG 751.9.1.2.4.2 Footing (Spread Footing and Pile Cap Footing) Joint Shear Reinforcement.
G1. Concrete BentsExpansion Device at End Bents (G1.1 and G1.1.1) (G1.1)
(G1.1.1)
(G1.2)
(G1.3)
(G1.5) Use for Non-Integral End Bents only.
(G1.6) Add to sheet showing the typical section thru wing detail.
(G1.7) Place with part plan of end bent, second F bar required for skewed bents.
(G1.7.1) Use for skewed bents. Place with plan of beam showing reinforcement and part plan of end bent, V bars not required with part plan of end bent.
(G1.8) Place with part plan of end bent.
P/S Structures (G1.9 and G1.9.1). place with part plan of end bent. (G1.9)
(G1.9.1) Use appropriate girder sheet number.
(G1.10) Use for steel structures without steel diaphragms at end bents.
(G1.11)
All Substructure Sheets with Anchor Bolts (G1.15A)
(G1.15B) Use unless only anchor bolt wells are preferred, i.e. uplift, congested reinforcement, etc.
(G1.16)
(G1.17) Use for P/S structures and for steel structures when the chair material is not the pay item material.
(G1.18) Use for structures with steel beam or girder pay items. Place below the substructure quantity box of all bents with chairs using the same pay item for (a) as used in Note G1.16.
(G1.19) Place with the other bent notes. Second sentence is required when the chair details are located with other bent details. Reinforcing steel shall be shifted to clear chairs. For details of chairs, see Sheet No. . Pile Cap Bents. (G1.20) Place with plan showing reinforcement.
Vertical Drains at End Bents. (G1.25) Place with part plan of end bent.
Bridge Approach Slab. (G1.30) Place with part plan of end bent.
(G1.40) Use the following note at all fixed intermediate bents on prestressed girder bridges with steps of 2" or more. Place with plan of beam.
(G1.41a) Use the following note when vertical column steel is hooked into the bent beam for seismic category A.
(G1.41b) Use the following note when vertical column steel is hooked into the bent beam for seismic category B, C or D.
(G1.42) Place the following note on plans when using Optional Section for Column-Web beam joints.
(G1.43) Place the following note on plans when you have adjoining twin bridges.
(G1.44) Use with column closed circular stirrup/tie bar detail.
(G1.45) Use when mechanical bar splices (MBS) are to be specified on the plans for column and drilled shaft vertical reinforcement.
REVISION REQUEST 4034!!! Only replace first part of 751.9.1 up to 751.9.1.1 !!! 751.9.1 Seismic Analysis and Design SpecificationsAll new or replacement bridges on the state system shall include seismic design and/or detailing to resist an expected seismic event per the Bridge Seismic Design Flowchart. For example, for a bridge in Seismic Design Categories A, B, C or D, complete seismic analysis or seismic detailing only may be determined as per “Bridge Seismic Design Flowchart”. Missouri is divided into four Seismic Design Categories. Most of the state is SDC A which requires minimal seismic design and/or detailing in accordance with SGS (Seismic Zone 1 of LRFD) and “Bridge Seismic Design Flowchart”. The other seismic design categories will require a greater amount of seismic design and/or detailing. For seismic detailing only: When AS is greater than 0.75 then use AS = 0.75 for abutment design where required per “Bridge Seismic Design Flowchart” and SEG 24-01 For complete seismic analysis: When AS is greater than 0.75 then use AS = 0.75 at zero second for seismic analysis and response spectrum curve. See Example 1_SDC_Response_Spectra. The other data points on the response spectrum curve shall not be modified. When existing bridges are identified as needing repairs or maintenance, a decision on whether to include seismic retrofitting in the scope of the project shall be determined per the “Bridge Seismic Retrofit Flowchart”, the extent of the rehabilitation work and the expected life of the bridge after the work. For example, if the bridge needs painting or deck patching, no retrofitting is recommended. However, redecking or widening the bridge indicates that MoDOT is planning to keep the bridge in the state system with an expected life of at least 30 more years. In these instances, the project core team should consider cost effective methods of retrofitting the existing bridge. Superstructure replacement requires a good substructure and the core team shall decide whether there is sufficient seismic capacity. Follow the design procedures for new or replacement bridges in forming logical comparisons and assessing risk in a rational determination of the scope of a superstructure replacement project specific to the substructure. For example, based on SPC and route, retrofit of the substructure could include seismic detailing only or a complete seismic analysis may be required determine sufficient seismic capacity. Economic analysis should be considered as part of the decision to re-use and retrofit, or re-build. Where practical, make end bents integral and eliminate expansion joints. Seismic isolation systems shall conform to AASHTO Guide Specifications for Seismic Isolation Design 4th Ed. 2023. Bridge seismic retrofit for widenings shall be in accordance with Bridge Seismic Retrofit Flowchart. Seismic details should only be considered for widenings where they can be practically implemented and where they can be uniformly implemented as not to create significant stress redistribution in the structure. When a complete seismic analysis is required for widenings the existing structure shall be retrofitted and the new structural elements shall be detailed to resist seismic demand.
751.40.3.2 Bent Cap Shear Strengthening using FRP Wrap
Fiber Reinforced Polymer (FRP) wrap may be used for Bent Cap Shear Strengthening. FRP wrap may also be used for seismic retrofit of existing columns, but that procedure is not discussed herein (see EPG 751.9.1 Seismic Analysis and Design Specifications). When to strengthen: When increased shear loading on an existing bent cap is required and a structural analysis shows insufficient bent cap shear resistance, bent cap shear strengthening is an option. An example of when strengthening a bent cap may be required: removing existing girder hinges and making girders continuous will draw significantly more force to the adjacent bent. An example of when strengthening a bent cap is not required: redecking a bridge where analysis shows that the existing bent cap cannot meet capacity for an HS20 truck loading, and the new deck is similar to the old deck and the existing beam is in good shape. How to strengthen: Using FRP systems for shear strengthening follows from the guidelines set forth in NCHRP Report 678, Design of FRP System for Strengthening Concrete Girders in Shear. The method of strengthening, using either discrete strips or continuous sheets, is made optional for the contractor in accordance with NCHRP Report 678. A Bridge Standard Drawing and Bridge Special Provision have been prepared for including this work on jobs. They can be revised to specify a preferred method of strengthening if desired, strips or continuous sheet. What condition of existing bent cap required for strengthening: If a cap is in poor shape where replacement should be considered, FRP should not be used. Otherwise, the cap beam can be repaired before applying FRP. Perform a minimum load check using (1.1DL + 0.75(LL+I))* on the existing cap beam to prevent catastrophic failure of the beam if the FRP fails (ACI 440.2R, Guide for the Design and Construction of Externally Bonded FRP, Sections 9.2 and 9.3.3). If the factored shear resistance of the cap beam is insufficient for meeting the factored minimum load check, then FRP strengthening should not be used.
Design force (net shear strength loading): Strengthening a bent cap requires determining the net factored shear loading that the cap beam must carry in excess of its unstrengthened factored shear capacity, or resistance. The FRP system is then designed by the manufacturer to meet this net factored shear load, or design force. The design force for a bent cap strengthening is calculated considering AASHTO LFD where the factored load is the standard Load Factor Group I load case. To determine design force that the FRP must carry alone, the factored strength of the bent cap, which is 0.85 x nominal strength according to LFD design, is subtracted out to give the net factored shear load that the FRP must resist by itself. NCHRP Report 678 is referenced in the special provisions as guidelines for the contractor and the manufacturer to follow. The report and its examples use AASHTO LRFD. Regardless, the load factor case is given and it is left to the manufacturer to provide for a satisfactory factor of safety based on their FRP system. Other References:
I5. Fiber Reinforced Polymer (FRP) Wrap – Intermediate Bent Column Strengthening for Seismic Details for Widening. Report following notes on Intermediate bent plan details.(I5.1)
(I5.2)
|